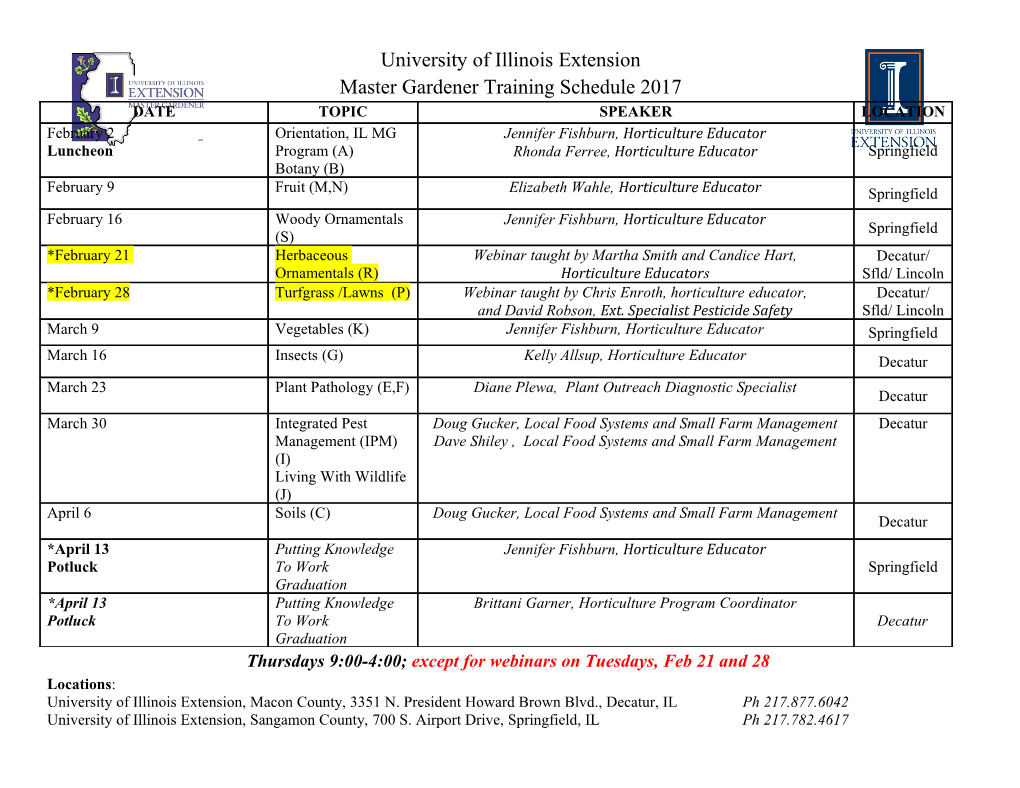
Correction BIOCHEMISTRY, CHEMISTRY Correction for “Transcriptome-wide profiling of multiple RNA modifications simultaneously at single-base resolution,” by Vahid Khoddami, Archana Yerra, Timothy L. Mosbruger, Aaron M. Fleming, Cynthia J. Burrows, and Bradley R. Cairns, which was first published March 14, 2019; 10.1073/pnas.1817334116 (Proc Natl Acad Sci USA 116:6784–6789). The authors note that the following statement should be added to the Acknowledgments: “This work was also supported by Grant R01 GM093099 from the NIH/National Institute of General Medical Sciences (to C.J.B.).” Published under the PNAS license. Published online April 22, 2019. www.pnas.org/cgi/doi/10.1073/pnas.1905628116 9136 | PNAS | April 30, 2019 | vol. 116 | no. 18 www.pnas.org Downloaded by guest on October 2, 2021 Transcriptome-wide profiling of multiple RNA modifications simultaneously at single-base resolution Vahid Khoddamia,b,c,1,2, Archana Yerrab,c,1, Timothy L. Mosbrugerd, Aaron M. Fleminge, Cynthia J. Burrowse,3, and Bradley R. Cairnsb,c,3 aDepartment of Cell Biology, Harvard Medical School, Boston, MA 02115; bHoward Hughes Medical Institute, University of Utah School of Medicine, Salt Lake City, UT 84112; cDepartment of Oncological Sciences, Huntsman Cancer Institute, University of Utah School of Medicine, Salt Lake City, UT 84112; dBioinformatics Shared Resource, Huntsman Cancer Institute, University of Utah School of Medicine, Salt Lake City, UT 84112; and eDepartment of Chemistry, University of Utah, Salt Lake City, UT 84112 Contributed by Cynthia J. Burrows, January 25, 2019 (sent for review October 9, 2018; reviewed by Juan D. Alfonzo, Thomas Carell, and Peter C. Dedon) The breadth and importance of RNA modifications are growing First, we provide the conceptual basis for sequencing/mismatch- rapidly as modified ribonucleotides can impact the sequence, struc- based detection of all three modifications (Fig. 1A) and an ex- ture, function, stability, and fate of RNAs and their interactions with ample tRNA (glycine) that illustrates modification clarity within other molecules. Therefore, knowing cellular RNA modifications at our HeLa cell dataset (Fig. 1 B and C, with multiple additional single-base resolution could provide important information regard- examples in SI Appendix, Figs. S1–S3). ing cell status and fate. A current major limitation is the lack of Detection of m5C in RNA (and DNA) relies on differential methods that allow the reproducible profiling of multiple modifi- sensitivity to bisulfite: unmethylated cytosine is efficiently de- cations simultaneously, transcriptome-wide and at single-base res- aminated by bisulfite ions converting cytosine to uridine, which is olution. Here we developed RBS-Seq, a modification of RNA bisulfite subsequently read as thymidine following desulfonation, RT- sequencing that enables the sensitive and simultaneous detection of PCR, and sequencing. In contrast, m5C resists bisulfite and re- m5C, Ψ,andm1A at single-base resolution transcriptome-wide. With A 5 1 mains cytosine after sequencing (15, 21) (Fig. 1 ). We improved RBS-Seq, m C and m A are accurately detected based on known prior m5C profiling methods by combining heat and the strong signature base mismatches and are detected here simultaneously chemical denaturant formamide, which improves RNA dena- along with Ψ sites that show a 1–2 base deletion. Structural anal- turation and bisulfite treatment (which preferentially modifies yses revealed the mechanism underlying the deletion signature, single-stranded RNA), providing a global C → T conversion which involves Ψ-monobisulfite adduction, heat-induced ribose ring opening, and Mg2+-assisted reorientation, causing base- Significance skipping during cDNA synthesis. Detection of each of these modifi- cations through a unique chemistry allows high-precision mapping of all three modifications within the same RNA molecule, enabling The field of RNA modification would be significantly advanced covariation studies. Application of RBS-Seq on HeLa RNA revealed by the development of sensitive, accurate, single-base resolu- almost all known m5C, m1A, and ψ sites in tRNAs and rRNAs and tion methods for profiling multiple common RNA modifications provided hundreds of new m5CandΨ sites in noncoding RNAs and in the same RNA molecule. Our work provides several advances mRNAs. However, our results diverge greatly from earlier work, toward that goal, including (i) quantitative methods for pro- suggesting ∼10-fold fewer m5C sites in noncoding and coding RNAs filing Ψ sites at true base-pair resolution transcriptome-wide, and the absence of substantial m1A in mRNAs. Taken together, the (ii) a chemical understanding of our observed Ψ-dependent 5 approaches and refined datasets in this work will greatly enable deletion signature, (iii) improved methods for profiling m C 1 future epitranscriptome studies. and m A, and (iv) a coupling of these methods for the simul- taneous detection of all three modifications in the same RNA. Together, the combinatorial ability and relative ease of exe- RNA modification | pseudouridine | RNA methylation | m1A | methyl cution provided by this procedure should greatly forward adenosine epitranscriptome studies involving these three very common RNA modifications. Covalent modifications of RNA are numerous (1), and transcriptome-wide profiling enables broad and systematic Author contributions: V.K. and B.R.C. designed research; V.K., A.Y., and A.M.F. performed analyses (2–4). Thus far, transcriptome-wide profiling has been research; V.K., A.Y., T.L.M., and A.M.F. contributed new reagents/analytic tools; V.K., A.Y., reported for a limited number of modifications including T.L.M., A.M.F., C.J.B., and B.R.C. analyzed data; and V.K., C.J.B., and B.R.C. wrote N6-methyladenosine (m6A), 5-methylcytosine (m5C), pseudour- the paper. idine (Ψ), and N1-methyladenosine (m1A) (5–14). However, Reviewers: J.D.A., Ohio State University; T.C., Ludwig Maximilians University; and P.C.D., Massachusetts Institute of Technology. profiling methods that provide sensitive and true single-base The authors declare no conflict of interest. resolution are currently available only for m5C (9, 14, 15) and 1 6 1 Ψ This open access article is distributed under Creative Commons Attribution-NonCommercial- m A (16); three of these (m A, m A, and ) have involved initial NoDerivatives License 4.0 (CC BY-NC-ND). 6 1 enrichment or detection via antibodies (for m Aorm A) (5, 6, 8, Data deposition: The data reported in this paper have been deposited in the Gene Ex- 10) or by techniques involving polymerase pausing/termination pression Omnibus (GEO) database, https://www.ncbi.nlm.nih.gov/geo (accession no. during reverse transcription (for m1A and Ψ) (7, 11–13, 17, 18). GSE90963). All custom computer scripts reported in this paper have been deposited in Recent single-base techniques for Ψ (19) rely on a bulky adduct GitHub, https://github.com/HuntsmanCancerInstitute/RBSSeqTools. formation before detection. Furthermore, although the current 1V.K. and A.Y. contributed equally to this work. methods for Ψ profiling are useful, most lack the sensitivity, 2Present address: Department of Stem Cells and Developmental Biology, Cell Science resolution, and technical ease needed for widespread adoption Research Center, Royan Institute for Stem Cell Biology and Technology, Academic – Center for Education, Culture and Research, 16635-148 Tehran, Iran. or straightforward candidate site validation (7, 11 13, 20). To 3 5 1 To whom correspondence may be addressed. Email: [email protected] or brad. provide simultaneous detection of m C, m A, and Ψ at single- [email protected]. base resolution transcriptome-wide from the same sample, we This article contains supporting information online at www.pnas.org/lookup/suppl/doi:10. developed a molecular approach and analysis pipelines for Ψ 1073/pnas.1817334116/-/DCSupplemental. 5 1 and improved sequencing-based methods for m C and m A. Published online March 14, 2019. 6784–6789 | PNAS | April 2, 2019 | vol. 116 | no. 14 www.pnas.org/cgi/doi/10.1073/pnas.1817334116 together), 143 sites in mRNAs (e.g., PTEN and HDGF; Figs. 2 A and B and 3A, SI Appendix, Fig. S2A, and Datasets S1 and S2), 14 pseudogenes, and 32 other noncoding RNAs. New sites within prominent mRNAs include PTEN, XRCC3, FANCA, RXRB, FGFR4, and EIF3B (Dataset S1). Importantly, examination of known/validated sites in tRNAs demonstrated that RBS-Seq has a dynamic range for m5C approaching 100% at single cytosines (e.g., C49 in Fig. 1C). Although our read numbers exceeded prior studies, our yield of 486 high-threshold candidate m5C sites in mRNA was far lower than the 10,275 sites reported previously (14), largely due to our more effective denaturation and de- amination/conversion, lowering false positives (SI Appendix,Figs. S10 and S11,andDataset S1). In keeping, more recent m5Cpro- filing in mouse ESCs that applies additional statistical and ana- lytical parameters to remove false-positives reports 266 sites in mRNA passing thresholds (24). Unlike m6A, m1A compromises A:T Watson–Crick base pairing, which pauses reverse transcriptase and elicits frequent nucleotide misincorporation, generating a single-base mismatch signature useful for m1A identification (8, 17, 25). As expected, in our NBS datasets from RBS-Seq, we indeed detected significant (FDR < 0.01) m1A-related mismatches at well-known m1A sites in non- coding RNAs [e.g., m1A-1322 in 28S rRNA (Fig. 2C and SI Ap- pendix, Fig. S2B) and m1A-58 in all tRNAs (Fig. 2D and Dataset S3)]. Unexpectedly, these mismatches were wholly absent or greatly diminished in our BS datasets (SI Appendix,TableS3), with tRNAs displaying the remarkable dynamic range of our method (∼90% for tRNAGly and tRNAThr;Fig.2D). Regarding the basis, BIOCHEMISTRY 1 6 Fig. 1. conversion of m AtomA (involving transfer of the methyl group RBS-Seq enables simultaneous base-pair resolution transcriptome- 1 6 wide mapping of m5C, m1A, and Ψ.(A) Schematic of reactivity of modified from N to the N position) occurs through a well-studied process nucleotides to bisulfite (Top) and the principle of simultaneous mapping of known as the Dimroth rearrangement (26) (SI Appendix,Fig.S12), modified nucleotides (Bottom).
Details
-
File Typepdf
-
Upload Time-
-
Content LanguagesEnglish
-
Upload UserAnonymous/Not logged-in
-
File Pages7 Page
-
File Size-