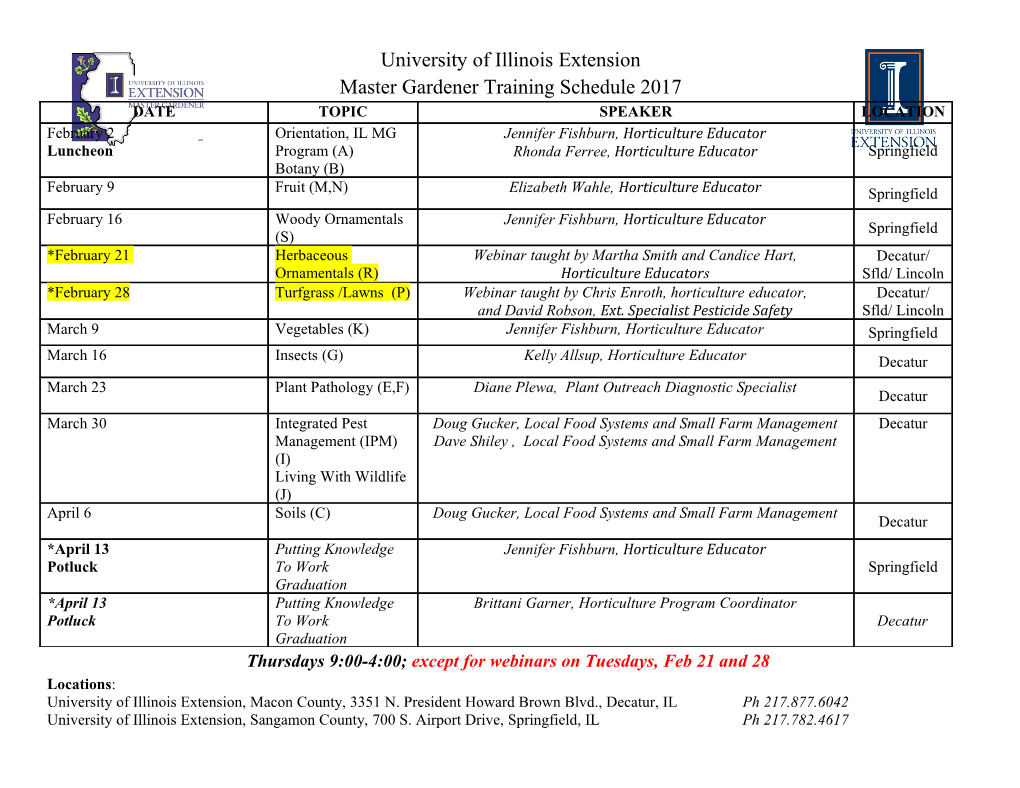
Analyst Accepted Manuscript This is an Accepted Manuscript, which has been through the Royal Society of Chemistry peer review process and has been accepted for publication. Accepted Manuscripts are published online shortly after acceptance, before technical editing, formatting and proof reading. Using this free service, authors can make their results available to the community, in citable form, before we publish the edited article. We will replace this Accepted Manuscript with the edited and formatted Advance Article as soon as it is available. You can find more information about Accepted Manuscripts in the Information for Authors. Please note that technical editing may introduce minor changes to the text and/or graphics, which may alter content. The journal’s standard Terms & Conditions and the Ethical guidelines still apply. In no event shall the Royal Society of Chemistry be held responsible for any errors or omissions in this Accepted Manuscript or any consequences arising from the use of any information it contains. www.rsc.org/analyst Page 1 of 43 Analyst 1 2 3 Submitted 05/07/2015 4 Revised 06/10/2015 5 6 7 Advances in Ion Mobility-Mass Spectrometry Instrumentation and 8 Techniques for Characterizing Structural Heterogeneity 9 10 Megan Maurer, 1 Gregory C. Donohoe, 1 and Stephen J. Valentine* 11 C. Eugene Bennett Department of Chemistry, West Virginia University, Morgantown, WV 26501 12 13 14 Abstract 15 16 17 Over the last decade, the field of ion mobility-mass spectrometry (IM-MS) has 18 19 experienced dramatic growth in its application toward ion structure characterization. Enabling 20 21 advances in instrumentation during this time period include improved conformation resolution 22 23 and ion sensitivity. Such advances have rendered IM-MS a powerful approach for 24 25 characterizing samples presenting a diverse array of ion structures. The structural 26 27 Manuscript 28 heterogeneity that can be interrogated by IM-MS techniques now ranges from samples 29 30 containing mixtures of small molecules exhibiting a variety of structural types to those containing 31 32 very large protein complexes and subcomplexes. In addition to this diversity, IM-MS techniques 33 34 have been used to probe spontaneous and induced structural transformations occurring in 35 36 solution or the gas phase. To support these measurement efforts, significant advances have 37 Accepted 38 been made in theoretical methods aimed at translating IM-MS data into structural information. 39 40 41 These efforts have ranged from providing more reliable trial structures for comparison to the 42 43 experimental measurements to dramatically reducing the time required to calculate collision 44 45 cross sections for such structures. In this short review, recent advances in developments in IM- Analyst 46 47 MS instrumentation, techniques, and theory are discussed with regard to their implications for 48 49 characterization of gas- and solution-phase structural heterogeneity. 50 51 52 53 1 54 Authors contributed equally to this work. 55 *e-mail: [email protected] 56 57 58 59 60 Analyst Page 2 of 43 1 2 3 Ion Mobility (IM) Resolution and Ion Sensitivity Improvements. Biomolecular ion 4 5 1, 2 6 structure characterization by ion mobility spectrometry (IMS) originated in the mid 1990s. For 7 8 such studies, the mobility measurement was used to determine an ion’s collision cross section 9 3 10 according to Equation 1. 11 12 ̊/̋ ̊/̋ ʚ̊̑ú ʛ л ̊ ̊ Ê ¡ ̐̏̉ ° ̊ 13 1) Ω = ̊̏ ʚÁ °ʛ̊/̋ ʢà + à ʣ ¨ ¬ ̋̐̌.̋ ª 14 ¥ ̉ 15 In Equation 1, t is the drift time or the drift region transit time of the ion. ze , m , and m are the 16 D I B 17 18 overall charge of the ion, the mass of the ion and the mass of the buffer gas, respectively. E 19 20 and L represent the electric field in the drift region and the length of the drift region and kB and T 21 22 are Boltzmann’s constant and the temperature of the buffer gas. P and N0 are the pressure of 23 24 the buffer gas and the neutral number density at STP, respectively. Ion conformation 25 26 information was originally obtained by comparing collision cross sections from experiments with 27 Manuscript 28 those calculated for theoretical three-dimensional structures. The theory behind such 29 30 4-6 31 comparisons developed nearly in concert with the experimental measurements. 32 33 It can be argued that improvement in comparisons between collision cross sections 34 35 obtained for computer-generated trial structures and experimentally determined values do not 36 37 scale directly with increased IM resolving power. For example, the reproducibility of low- Accepted 38 39 resolution mobility measurements (typically ± 2%) often exceeds the variability in ion structure 40 41 size observed in extended molecular dynamics simulations (MDS) for matching low-energy 42 43 44 conformations. However, for the purpose of characterizing gas- or solution-phase conformer 45 Analyst 46 heterogeneity, higher resolving power in the mobility dimension significantly enhances structural 47 48 studies. Such enhancements range from the determination of the degree of co-existing solution 49 50 structures for biomolecular species to the determination of structural heterogeneity resulting 51 52 from induced or spontaneous conformational transformations in the gas- and/or solution- 53 54 55 phases. As an example of the utility of increased mobility resolving power for structure 56 57 ensemble characterization, consider Figure 1. Multidimensional IM separations showed that 58 59 60 Page 3 of 43 Analyst 1 2 3 conformational types of gas-phase protein ions resolved by a single IM measurement step 4 5 7 6 actually consist of many separate, unresolved conformers exhibiting unique mobilities. Another 7 8 example is the need to adequately resolve the structural heterogeneity associated with complex 9 8-10 10 mixtures such as those encountered in ‘omics investigations. Indeed, such requirements 11 12 have in part influenced IM-MS instrumentation development focused on improving the resolution 13 14 of the mobility-based separation. 15 16 11 The resolving power (R) of a traditional IM measurement is described by Equation 2. 17 18 19 ¨¡Ð» ̊/̋ ® = ʠ ʡ 2) 20 ̊̏ Á° ÂÄ ̋ 21 22 Here, R represents the ratio of the ion’s drift time ( tD) to the width of the peak at half-maximum 23 24 height. Shortly after the application of IM-MS techniques for the characterization of 25 26 biomolecular ion structure, researchers began to explore the development of instrumentation 27 Manuscript 28 that would exploit instrumental parameter settings (Equation 2) to achieve high-resolution 29 30 12, 13 31 measurements for biological ions. Although, high resolution measurements were reported 32 33 nearly 20 years ago, maximizing R by changing instrument geometry and operational settings 34 35 (L, E, and T from Equation 2), was shown to reach a point of diminishing returns resulting from 36 37 unmanageable operational conditions and spatial requirements. These challenges provided the Accepted 38 39 impetus for developing different strategies for achieving high resolution IM separation 40 41 capabilities. Notably, Equations 1 and 2 are applicable to measurements performed on 42 43 44 constant-field drift tubes. However, because collision cross sections can be obtained from other 45 Analyst 46 mobility measurements, the discussion below is not limited to instrumentation employing 47 48 traditional drift tube geometries. Although much has been accomplished in the area of 49 50 resolution improvement for the technique of field-assymetric ion mobility spectrometry (FAIMS) 51 52 [or differential mobility spectrometry (DMS)], 14 this technique is not discussed here as the 53 54 55 purpose of this review is to describe techniques for which collision cross sections can be 56 57 determined to provide a description of structural heterogeneity. 58 59 60 Analyst Page 4 of 43 1 2 3 Recently, established and new mobility-based measurements were shown to 4 5 6 significantly extend the achievable resolving power of mobility measurements in IM-MS 7 8 instrumentation. Although traveling wave IM (TWIM) measurements were demonstrated more 9 15 10 than a decade ago, recent improvements in electric field application and pressure settings 11 12 yielded a four-fold improvement in mobility resolving power. 16 Again, with regard to established 13 14 technologies, de la Mora recently showed the utility of high-resolution differential mobility 15 16 analysis in distinguishing conformations of charge-reduced protein and protein complex ions. 17 17 18 19 Within the last few years, new high-resolution IM techniques were demonstrated with MS 20 21 analyses. One example was Trapped Ion Mobility Spectrometry (TIMS) which utilized a flow of 22 18 23 gas applied along the ion separation axis, RF confining fields, and a variable DC gradient. By 24 25 scanning the DC gradient, ions of different mobilities could be sequentially removed from the ion 26 27 trap and detected. Recently Park and coworkers demonstrated high resolution measurements Manuscript 28 29 on a TIMS device. 19 Resolving power as high as ~200 were obtained for the analysis of 30 31 32 peptide ions. Overtone Mobility Spectrometry (OMS) was also presented as an alternative 33 20 34 mobility-based separation approach. As described OMS utilized periodic ion gates equally 35 36 spaced along the mobility separation axis which served to allow only the transmission of ions of 37 Accepted 38 select mobilities (i.e., ions that traversed intervening regions of a segmented drift tube in the 39 40 gate time period). Recently, Clemmer and coworkers utilized an OMS-type separation with a 41 42 circular drift tube to achieve resolving power values in excess of 1000. 21 These represent the 43 44 45 highest resolving power measurements to date for mobility-based separations.
Details
-
File Typepdf
-
Upload Time-
-
Content LanguagesEnglish
-
Upload UserAnonymous/Not logged-in
-
File Pages44 Page
-
File Size-