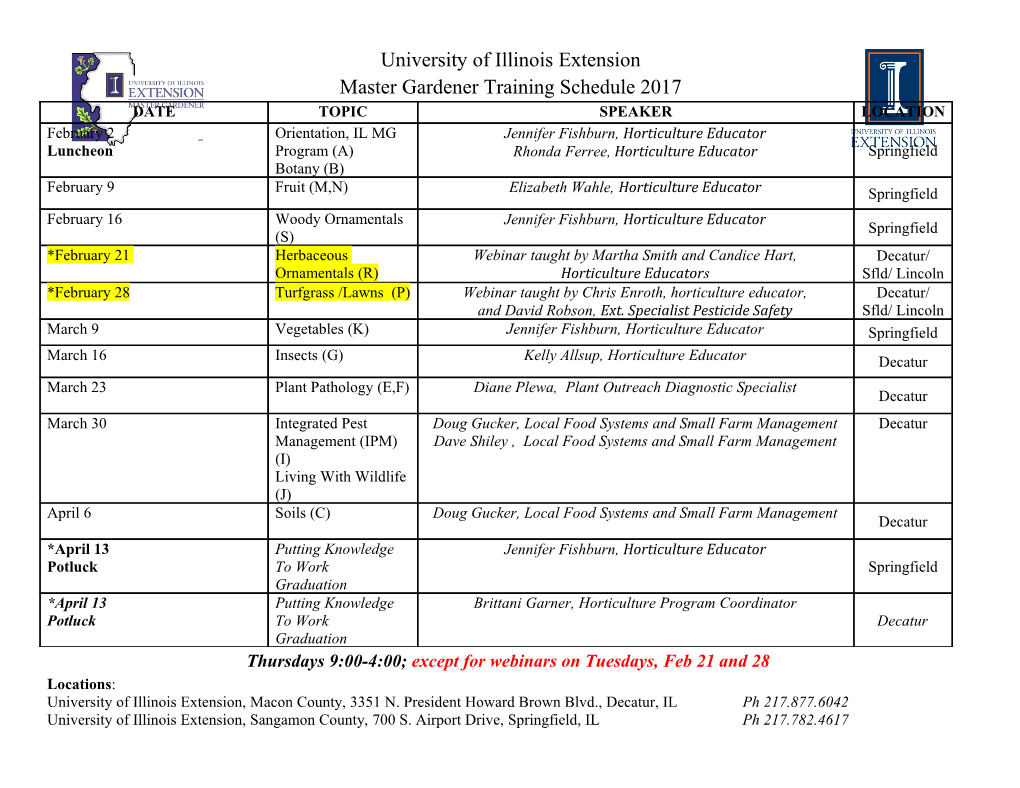
Geomorphology 293 (2017) 227–239 Contents lists available at ScienceDirect Geomorphology journal homepage: www.elsevier.com/locate/geomorph A 184-year record of river meander migration from tree rings, aerial MARK imagery, and cross sections ⁎ Derek M. Schooka, , Sara L. Rathburna, Jonathan M. Friedmanb, J. Marshall Wolfc a Department of Geosciences, Colorado State University, 1482 Campus Delivery, Fort Collins, CO 80523, USA b U.S. Geological Survey, Fort Collins Science Center, 2150 Centre Avenue, Building C, Fort Collins, CO 80526, USA c Department of Ecosystem Science and Sustainability, Colorado State University, 1476 Campus Delivery, Fort Collins, CO 80523, USA ARTICLE INFO ABSTRACT Keywords: Channel migration is the primary mechanism of floodplain turnover in meandering rivers and is essential to the Channel migration persistence of riparian ecosystems. Channel migration is driven by river flows, but short-term records cannot Meandering river disentangle the effects of land use, flow diversion, past floods, and climate change. We used three data sets to Aerial photography quantify nearly two centuries of channel migration on the Powder River in Montana. The most precise data set Dendrochronology came from channel cross sections measured an average of 21 times from 1975 to 2014. We then extended spatial Channel cross section and temporal scales of analysis using aerial photographs (1939–2013) and by aging plains cottonwoods along transects (1830–2014). Migration rates calculated from overlapping periods across data sets mostly revealed cross-method consistency. Data set integration revealed that migration rates have declined since peaking at 5 m/ year in the two decades after the extreme 1923 flood (3000 m3/s). Averaged over the duration of each data set, cross section channel migration occurred at 0.81 m/year, compared to 1.52 m/year for the medium-length air photo record and 1.62 m/year for the lengthy cottonwood record. Powder River peak annual flows decreased by 48% (201 vs. 104 m3/s) after the largest flood of the post-1930 gaged record (930 m3/s in 1978). Declining peak discharges led to a 53% reduction in channel width and a 29% increase in sinuosity over the 1939–2013 air photo record. Changes in planform geometry and reductions in channel migration make calculations of flood- plain turnover rates dependent on the period of analysis. We found that the intensively studied last four decades do not represent the past two centuries. 1. Introduction from more subtle changes in land cover (Costa et al., 2003), irrigation extraction (Johnson, 1994), and climate (Favaro and Lamoureux, Flooding, sediment redistribution, and recycling of land are re- 2015). Climate change has modified the timing and magnitude of flood quired for the persistence of healthy river ecosystems (Gurnell et al., peaks, especially in snowmelt-driven rivers (Stewart et al., 2005; Clow, 2012; Meitzen et al., 2013). River flows not only transport in-channel 2010). Land use and land cover changes can increase (Costa et al., sediment, but their lateral channel migration also destroys and creates 2003) or decrease (Li et al., 2009) flood magnitudes. Channel migration land (Hickin and Nanson, 1975; Wohl et al., 2015). Channel migration can be directly suppressed through bank stabilization (Florsheim et al., rates vary between 0 and > 100 m/year across reaches, rivers, and 2008) or indirectly through species invasion (Cadol et al., 2011), flow hydroclimatic periods (Hooke, 1980; Lawler, 1993; Hudson and Kesel, regulation, and water extraction (Fremier et al., 2014). Any of these 2000). Modification to fluvial disturbance regimes can transition modifications can alter channel migration produced by point bar floodplains into upland communities with lower biodiversity (Merritt growth and cutbank recession (Parker et al., 2011; van de Lageweg and Cooper, 2000; Johnson et al., 2012). Even minor changes to water et al., 2014). and sediment regimes may have significant effects, which makes free- Channel migration is somewhat predictable (Howard and Knutson, flowing meandering rivers excellent locations to detect environmental 1984; Luchi et al., 2007), but ultimately it is sufficiently complex to changes (Erskine et al., 1992; Palmer et al., 2008). prevent accurate prediction of changes in a given meander (Nanson and Several conditions can alter the physical processes responsible for Hickin, 1983; Nanson, 1986; Hooke, 2007; Güneralp et al., 2012). channel migration. Damming dramatically alters fluvial processes and Channel gradient, meander curvature, bank resistance, and flow history landforms (Brandt, 2000; Nilsson et al., 2005), but shifts also result all affect the sensitivity of river meanders. Flow and geomorphic ⁎ Corresponding author. E-mail address: [email protected] (D.M. Schook). http://dx.doi.org/10.1016/j.geomorph.2017.06.001 Received 22 December 2016; Received in revised form 2 June 2017; Accepted 2 June 2017 Available online 06 June 2017 0169-555X/ © 2017 Elsevier B.V. All rights reserved. D.M. Schook et al. Geomorphology 293 (2017) 227–239 histories affect channel response to subsequent flows (Schumm, 1973; flows have been removed for uses such as irrigation (Chase, 2013), the Moody et al., 1999). For example, bank erosion may be affected by decrease in mean annual flow from 1931 to 2010 was not significant deposits from previous floods (Nanson and Hickin, 1983; Nanson, (Schook et al., 2016). Water development has had little impact on peak 1986), and extreme floods may incite channel responses that last sev- flows (Chase, 2013). eral decades. Because characterizing different meanders is difficult, Powder River floods are produced in four main ways (Moody et al., assessing meander migration at the reach scale is useful for character- 2002; Moody and Meade, 2014). First, low-elevation ice jam floods izing channel migration. Additionally, because of the time lags affecting occur between late February and early April when the southern, up- channel responses to dynamic flows and large floods, channel migration stream reaches of the river thaw before downstream reaches. These should be considered over a range of timescales. floods are spatially limited. Second, Bighorn Mountains high elevation Diverse research approaches have been used to study channel mi- snowmelt in May–June creates the peak annual flow in 60% of years gration, including repeat planimetric and cross sectional surveys, (Schook et al., 2016). These snowmelt floods can be augmented by structure from motion (Fonstad et al., 2013), terrestrial laser scanning spring rain, as occurred in 1978, to produce the largest peak discharge (Milan et al., 2007), photogrammetry (Lawler, 1993), and numerical (930 m3/s) in the continuous gage record. Third, flash floods from modeling (Larsen et al., 2006). The data sets generated from these convective rainstorms occasionally produce short-duration high flows approaches are usually collected over short timescales (years), leaving in spring, summer, and fall. Fourth, frontal storms can cause autumn high uncertainty in characterization of migration at the century-to- floods. The largest documented flood was in 1923 and preceded the millennial timescales (Hughes, 1997). Approaches addressing multi- gage record. It peaked at an estimated 3000 m3/s and was caused by a century timescales include radio carbon dating (Knox, 2000; Erskine slow-moving September cold front that stalled over the region for et al., 2012) and optically stimulated luminescence (Hobo et al., 2014), several days (Grover, 1925). but both techniques generally suffer from low spatial extent and low Since 1975, Moody and Meade (1990) have monitored 24 perma- temporal resolution. Intermediate length (101–102 years) data sets nent channel cross sections along the Powder River between Moorhead might be best for characterizing channel migration. These include and Broadus, MT, just north of the Wyoming-Montana border. Our multidecade channel cross section measurements, which have revealed study reach is a subset of the Moody and Meade (1990) study area mechanisms of floodplain formation (Pizzuto, 1994; Moody and Meade, between PR130 and PR194, a valley distance of 37 km and a 2013 river 2008) and meander evolution (Hooke, 2007). Aerial photographs pro- distance of 75 km (Fig. 1). The channel bed is composed of sand and vide data sets with longer timescales that extend up to 80 years. gravel (Hembree et al., 1952), while the point bars and floodplain are Dendrochronology (tree ring science) can offer an approach cov- ~80% silt-fine sand (Moody and Meade, 2008). ering longer timescales than channel cross sections and air photos, which is possible when floodplain trees establish predictably in re- 2.2. Floodplain forests sponse to channel migration (Merigliano et al., 2013). Cottonwood trees (Populus spp.) grow in floodplains and can provide a living record Plains cottonwood (Populus deltoides ssp. monilifera) is the most of centuries of channel changes (Hickin and Nanson, 1975; Edmondson common riparian tree across the western USA (Friedman et al., 2005), et al., 2014). Cottonwoods are widely distributed on floodplains in the including along the lower Powder River. Additional woody vegetation western USA (Friedman et al., 2005). Their propensity to establish soon includes native sandbar willow (Salix exigua) and exotics Russian olive after a point bar surface is created means that channel migration can be (Elaeagnus angustifolia) and tamarisk (Tamarix ramosissima). Cotton- traced using their ages (Cooper et al., 2003; Stella et al., 2011;
Details
-
File Typepdf
-
Upload Time-
-
Content LanguagesEnglish
-
Upload UserAnonymous/Not logged-in
-
File Pages13 Page
-
File Size-