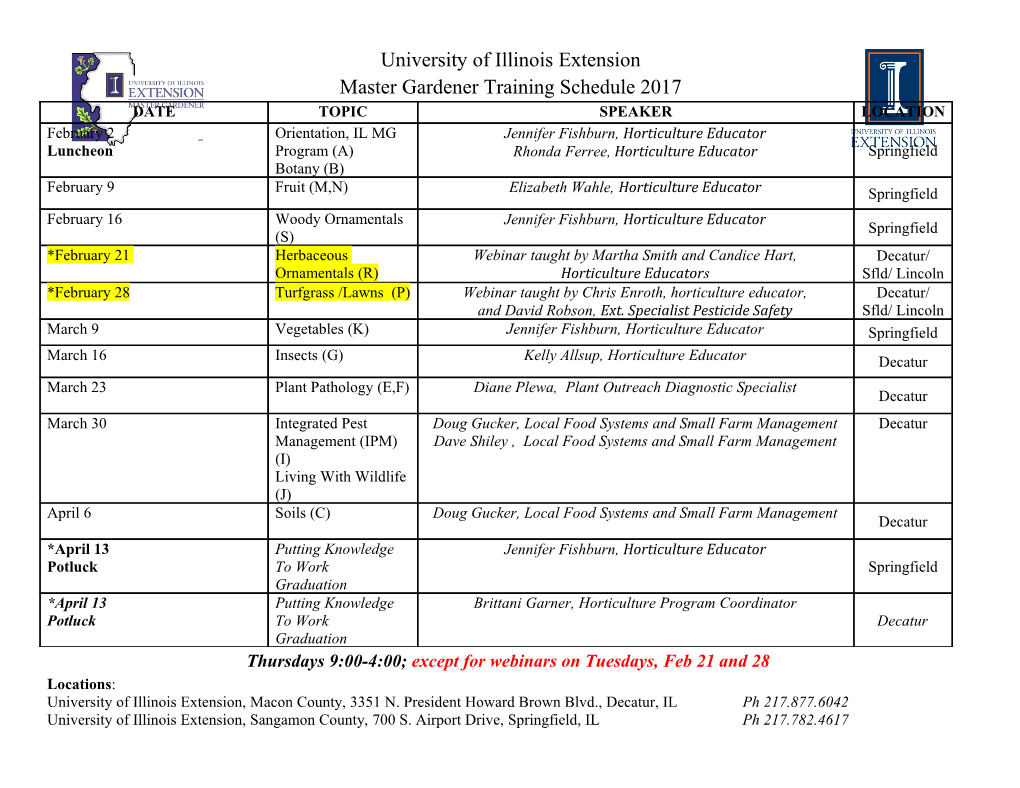
bioRxiv preprint doi: https://doi.org/10.1101/2020.02.20.957654; this version posted February 20, 2020. The copyright holder for this preprint (which was not certified by peer review) is the author/funder, who has granted bioRxiv a license to display the preprint in perpetuity. It is made available under aCC-BY-NC-ND 4.0 International license. 1 Modulation of bacterial multicellularity via spatiotemporal polysaccharide secretion 2 3 4 Salim T. Islam1,2,3†*, Israel Vergara Alvarez3†, Fares Saïdi1,2,3†, Annick Giuseppi3, Evgeny 5 Vinogradov4, Castrese Morrone3, Gael Brasseur3, Gaurav Sharma5,6, Anaïs Benarouche7, Jean- 6 Luc Bridot7, Gokulakrishnan Ravicoularamin1, Alain Cagna7, Charles Gauthier1, Mitchell 7 Singer5, Henri-Pierre Fierobe3, Tâm Mignot3, Emilia M. F. Mauriello3* 8 9 1 Armand Frappier Health & Biotechnology Research Centre, Institut National de la Recherche 10 Scientifique, Université du Québec, Institut Pasteur International Network, Laval, QC, Canada 11 2 PROTEO, the Quebec Network for Research on Protein Function, Engineering, and 12 Applications, Université Laval, Quebec, QC, Canada 13 3 Laboratoire de Chimie Bactérienne, CNRS - Université Aix-Marseille UMR7283, Institut de 14 Microbiologie de la Méditerranée, Marseille, France 15 4 National Research Council, 100 Sussex Dr., Ottawa, Ontario, Canada 16 5 Department of Microbiology and Molecular Genetics, University of California – Davis, Davis, 17 CA, USA 18 6 Institute of Bioinformatics and Applied Biotechnology, Electronic City, Bengaluru, Karnataka, 19 560100, India 20 7 Teclis Scientific, Civrieux d’Azergue, France 21 22 †equal contribution 23 24 25 *co-corresponding authors 26 27 Salim T. Islam Emilia M. F. Mauriello 28 E-mail: [email protected] E-mail: [email protected] 29 Phone: (+1) 450-687-5010 ext. 8897 Phone: (+33) 04 91 16 43 21 Page 1 of 52 bioRxiv preprint doi: https://doi.org/10.1101/2020.02.20.957654; this version posted February 20, 2020. The copyright holder for this preprint (which was not certified by peer review) is the author/funder, who has granted bioRxiv a license to display the preprint in perpetuity. It is made available under aCC-BY-NC-ND 4.0 International license. 30 ABSTRACT: 31 The development of multicellularity is a key evolutionary transition allowing for 32 differentiation of physiological functions across a cell population that confers survival benefits; 33 among unicellular bacteria, this can lead to complex developmental behaviours and the 34 formation of higher-order community structures. Herein, we demonstrate that in the social δ- 35 proteobacterium Myxococcus xanthus, the secretion of a novel secreted biosurfactant 36 polysaccharide (BPS) is temporally and spatially modulated within communities, mediating 37 swarm migration as well as the formation of multicellular swarm biofilms and fruiting bodies. 38 BPS is a type IV pilus-inhibited acidic polymer built of randomly-acetylated β-linked 39 tetrasaccharide repeats. Both BPS and the “shared good” EPS are produced by dedicated 40 Wzx/Wzy-dependent polysaccharide assembly pathways distinct from that responsible for spore 41 coat assembly. To our knowledge, such pathways have never-before been explicitly shown to 42 synthesize a biosurfactant. Together, these data reveal the central role of secreted 43 polysaccharides in the intricate behaviours coordinating bacterial multicellularity. Page 2 of 52 bioRxiv preprint doi: https://doi.org/10.1101/2020.02.20.957654; this version posted February 20, 2020. The copyright holder for this preprint (which was not certified by peer review) is the author/funder, who has granted bioRxiv a license to display the preprint in perpetuity. It is made available under aCC-BY-NC-ND 4.0 International license. 44 INTRODUCTION: 45 Multicellularity is denoted by the differentiation of physiological functions across a 46 contiguous cell population, with its development regarded as a key evolutionary transition [1]. 47 To attain this level of organizational complexity, cells generally must be able to proliferate, 48 specialize, communicate, interact, and move, with these behaviours promoting an increase in the 49 size of a cell collective and the development of higher-order structures [2]. Though typically 50 associated with metazoan organisms, multicellular physiology is also displayed by bacteria, with 51 the best-studied examples being the formation of biofilms and fruiting bodies [3-6]. 52 Secreted long-chain polysaccharides are an important mediator of multicellularity as they 53 serve to retain and organize cells as well as to physically and biochemically buffer the 54 community within the context of an extracellular matrix [7], thus enhancing survival and fitness. 55 Monospecies bacterial biofilms have thus been intensively studied with respect to their effects on 56 inter-cell communication, leading to differences in gene regulation and changes in matrix 57 polysaccharide production. However, in-depth knowledge of the mechanisms used by bacteria to 58 modulate multicellular physiology in such communities is limited. 59 Due to its complex social predatory lifecycle, the Gram-negative δ-proteobacterium 60 Myxococcus xanthus has emerged as a leading model system in which to simultaneously study 61 multiple factors contributing to organizational complexity. This soil bacterium is capable of 62 saprophytic feeding on products derived from predation of other bacteria [8]. Two forms of 63 motility are required for this complex physiology: type IV pilus (T4P)-dependent group (i.e. 64 “social” [S]) motility [9, 10] on soft surfaces, and single-cell gliding (i.e. “adventurous” [A]) 65 motility on hard surfaces mediated by directed transport and substratum coupling of the Agl–Glt 66 trans-envelope complex [11, 12]. Upon local nutrient depletion, cells initiate a developmental Page 3 of 52 bioRxiv preprint doi: https://doi.org/10.1101/2020.02.20.957654; this version posted February 20, 2020. The copyright holder for this preprint (which was not certified by peer review) is the author/funder, who has granted bioRxiv a license to display the preprint in perpetuity. It is made available under aCC-BY-NC-ND 4.0 International license. 67 cycle resulting in aggregation and fruiting body formation within 72 h, generating three 68 differentiated cell subpopulations: (i) cells that form desiccation-resistant myxospores in the 69 centre of the fruiting body, (ii) those that remain at the base of the fruiting body, termed 70 “peripheral rods”, and (iii) forager cells that continue their outward motility away from the 71 fruiting body [13]. 72 M. xanthus produces several known long-chain polysaccharides that are central to its 73 complex lifecycle. In addition to the O-antigen polymer that caps its LPS and is implicated in 74 motility [14-16], M. xanthus biosynthesizes a poorly characterized “slime” polysaccharide that 75 facilitates adhesion of the Glt gliding motility complex proteins to the substratum, and is 76 deposited in trails behind surface-gliding cells [17, 18]. Exopolysaccharide (EPS) is a specific 77 secreted polymer of this bacterium that is important for T4P-dependent swarm spreading; it is 78 also crucial for biofilm formation as it constitutes a large portion of the extracellular matrix in 79 stationary M. xanthus biofilms and connects cells via a network of fibrils [19-21]. The 80 production of EPS requires the presence of a T4P [22], which affects the Dif chemosensory 81 pathway (reviewed elsewhere [13]). Finally, cells undergoing sporulation synthesize the major 82 spore coat (MASC) polymer that surrounds myxospores [23, 24]. 83 The most widespread polysaccharide biosynthesis paradigm is the flippase/polymerase 84 (Wzx/Wzy)-dependent pathway [25]. It is used by Gram-negative and Gram-positive bacteria as 85 well as Archaea to produce a wide range of secreted and/or cell surface-associated bacterial 86 polymers [26] including capsular polysaccharide, adhesive hold-fast polymer, spore-coat 87 polymer, O-antigen, and exopolysaccharide [27, 28]. Wzx/Wzy-dependent polysaccharide 88 assembly is a complex process [29] involving a suite of integral inner-membrane (IM) proteins 89 containing multiple α-helical transmembrane segments (TMS) [30]. At the cytoplasmic leaflet of Page 4 of 52 bioRxiv preprint doi: https://doi.org/10.1101/2020.02.20.957654; this version posted February 20, 2020. The copyright holder for this preprint (which was not certified by peer review) is the author/funder, who has granted bioRxiv a license to display the preprint in perpetuity. It is made available under aCC-BY-NC-ND 4.0 International license. 90 the IM, individual polysaccharide repeat units are built on an undecaprenyl pyrophosphate 91 (UndPP) carrier. UndPP-linked repeats are then translocated across the IM by the Wzx flippase 92 [31, 32] via a putative antiport mechanism [33, 34]. Defects in this step, resulting in a buildup of 93 UndPP-linked repeat units for a given pathway, can have adverse effects on cell growth as well 94 as polysaccharide synthesis by other pathways in the same cell, all dependent on UndPP-linked 95 sugars [35]. Once in the periplasmic leaflet of the IM, repeat units are joined together by two 96 key periplasmic loops of the Wzy polymerase [36-39], resulting in polymer extension at the 97 reducing terminus of the growing chain [40]. Repeat-polymerization defects in a given pathway 98 requiring UndPP may also affect other pathways requiring UndPP due to sequestration of the 99 cellular UndPP pool. Associated polysaccharide co-polymerase
Details
-
File Typepdf
-
Upload Time-
-
Content LanguagesEnglish
-
Upload UserAnonymous/Not logged-in
-
File Pages59 Page
-
File Size-