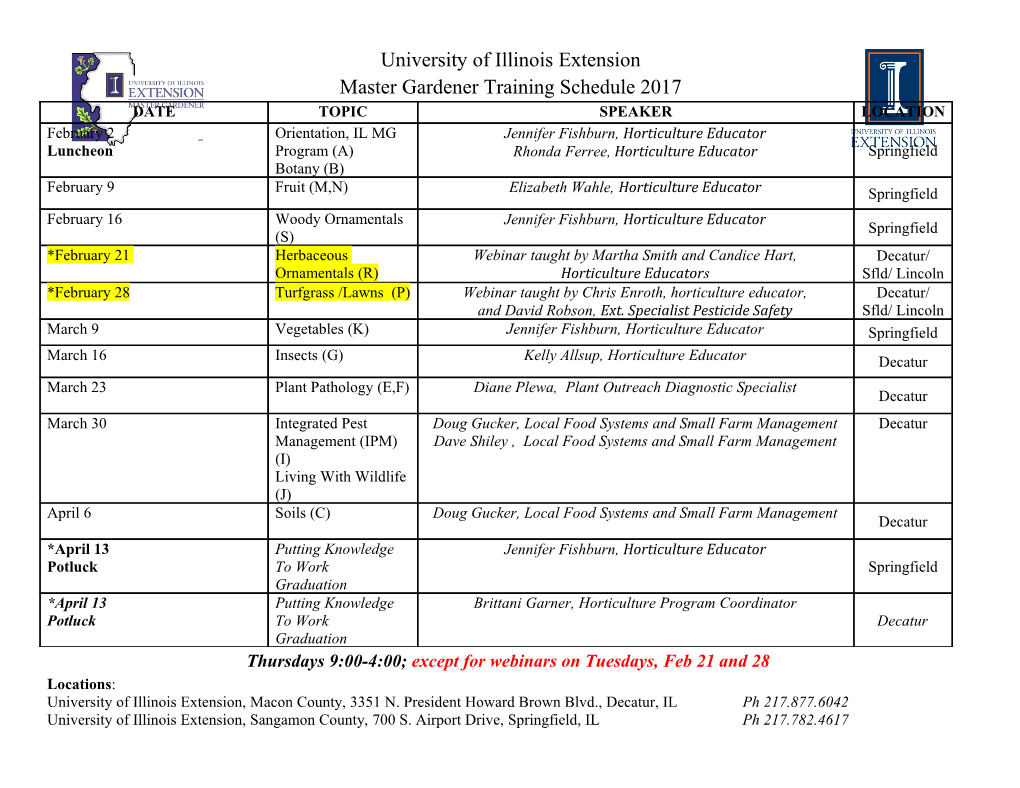
PHYSICS 116A Homework 9 Solutions 1. Boas, problem 3.12–4. Find the equations of the following conic, 2 2 3x + 8xy 3y = 8 , (1) − relative to the principal axes. In matrix form, Eq. (1) can be written as: 3 4 x (x y) = 8 . 4 3 y − I could work out the eigenvalues by solving the characteristic equation. But, in this case I can work them out by inspection by noting that for the matrix 3 4 M = , 4 3 − we have λ1 + λ2 = Tr M = 0 , λ1λ2 = det M = 25 . − It immediately follows that the two eigenvalues are λ1 = 5 and λ2 = 5. Next, we compute the − eigenvectors. 3 4 x x = 5 4 3 y y − yields one independent relation, x = 2y. Thus, the normalized eigenvector is x 1 2 = . y √5 1 λ=5 Since M is a real symmetric matrix, the two eigenvectors are orthogonal. It follows that the second normalized eigenvector is: x 1 1 = − . y − √5 2 λ= 5 The two eigenvectors form the columns of the diagonalizing matrix, 1 2 1 C = − . (2) √5 1 2 Since the eigenvectors making up the columns of C are real orthonormal vectors, it follows that C is a real orthogonal matrix, which satisfies C−1 = CT. As a check, we make sure that C−1MC is diagonal. −1 1 2 1 3 4 2 1 1 2 1 10 5 5 0 C MC = − = = . 5 1 2 4 3 1 2 5 1 2 5 10 0 5 − − − − − 1 Following eq. (12.3) on p. 162 of Boas, x x′ = C , y y′ where (x′ y′) are the principal axes. Hence, using CT = C−1, it follows that: ′ ′ x ′ ′ T x ′ ′ −1 x (x y) M = x y C MC = x y C MC y y′ y′ ′ ′ ′ 5 0 x ′ 2 ′ 2 = x y ′ = 5(x y ) , (3) 0 5 y − − That is, relative to the principal axes, Eq. (1) takes the form ′ 2 ′ 2 5(x y ) = 8 − 2. Boas, problem 3.12–16. Find the characteristic frequencies and the characteristic modes of vibra- tion for the system of masses and springs as in Figure 12.1 on p. 165 of Boas, for the following array: 4k, m, 2k, m, k. Following the discussion of Example 3 on pp. 165–166 of Boas, the total potential energy is 2 2 2 2 2 V = 1 (4k)x + 1 (2k)(x y) + 1 ky = k 3x 2xy + 3 y . 2 2 − 2 − 2 The equations of motion are: mx¨ = ∂V/∂x = 6kx + 2ky, − − (4) (my¨ = ∂V/∂y = 2kx 3ky, − − 2 2 2 2 wherex ¨ d x/dt andy ¨ d y/dt . Assuming solutions of the form x = x0eiωt and y = y0eiωt, ≡ ≡ it follows that 2 2 x¨ = ω x andy ¨ = ω y. − − Substituting these results into Eq. (4) yields: mω2x = ∂V/∂x = 6kx + 2ky, − 2 − − ( mω y = ∂V/∂y = 2kx 3ky. − − − In matrix form, these equations are: 2 6 2 x x mω − = λ , with λ . (5) 2 3 y y ≡ k − But the coefficients matrix above is precisely the matrix whose eigenvalue problem was solved in Qu. (10) on homework set 8. Thus, the eigenvalues are λ = 7 and λ = 2 with corresponding normalized eigenvectors x 1 2 x 1 1 = − and = . y √5 1 y √5 2 λ=7 λ=2 2 Using Eq. (5), ω =(λk/m)1/2. Thus, there are two characteristic frequencies, 7k 2k ω1 = and ω2 = . r m r m The corresponding characteristic modes of vibrations are identified by the unnormalized eigenvec- tors, x 2 x 1 = c1 − and = c2 , y 1 y 2 ω1 ω2 where c1 and c2 are non-zero constants. These modes describe displacements which oscillate at a single frequency. The general solution to the equations of motion is a linear combination of these two modes and so exhibits oscillations at two frequencies. 3. Boas, problem 10.2–7. Following eqs. (2.14)–(2.17) on pp. 500–501 of Boas, show that the direct product of a vector and a third-rank tensor is a fourth-rank tensor. Also, show that the direct product of two second-rank tensors is a fourth-rank tensor. Generalize these results to show that the direct product of two tensors of ranks m and n is a tensor of rank m + n. Here, I follow the notation of Boas, and use aij for the matrix elements of the rotation matrix (rather than Rij which was used in class). An nth rank Cartesian tensor is defined according to the transformation law of its components with respect to rotations (either proper or improper). In particular, ′ T ··· = a a a a n n T ··· n , (6) i1i2i3 in i1j1 i2j2 i3j3 ··· i j i1i2i3 i where the Einstein summation convention is being used to sum over the repeated indices j1,j2,j3,...,jn. Thus, if I multiply an nth rank Cartesian tensor by an mth rank Cartesian tensor, where the trans- formation property of the latter is ′ S ··· = a a a a S ··· , (7) k1k2k3 km k1ℓ1 k2ℓ2 k3ℓ3 ··· kmℓm ℓ1ℓ2ℓ3 ℓm then one obtains a new tensor with n + m indices: P ··· ··· T ··· n S ··· . i1i2i3 ink1k2k3 km ≡ i1i2i3 i k1k2k3 km This is called a direct product of two tensors. Relative to the rotated coordinate system, ′ ′ ′ Pi1i2i3···ink1k2k3···km = Ti1i2i3···in Sk1k2k3···km . Hence, the transformation law for the product tensor P follows immediately from Eqs. (6) and (7): ′ P ··· ··· =(a a a a n n )(a a a a )P ··· ··· , i1i2i3 ink1k2k3 km i1j1 i2j2 i3j3 ··· i j k1ℓ1 k2ℓ2 k3ℓ3 ··· kmℓm j1j2j3 jnℓ1ℓ2ℓ3 ℓm which is the correct transformation law for a tensor of rank m + n. Specializing the above proof to the direct product of a vector and a third-rank tensor and to the direct product of two second-rank tensors is immediate, and I will not write out the proof explicitly. 3 4. Boas, problem 10.3–3. Show that the contracted tensor Tiik is a first-rank tensor, that is, a vector. The transformation law of a third-rank tensor Tijk due to the rotation of the coordinate system is given by: ′ Tijk = aiℓajmaknTℓmn , (8) where I am using the Einstein summation convention to sum implicitly over the repeated indices ℓ, m and n. Now, set i = j in Eq. (8) and sum over j. One obtains ′ Tiik = aiℓaimaknTℓmn , (9) T Recall that A =[aij] is an orthogonal matrix, which means that A A = I. In component form we have: T (A A)jk = aijaik = δjk . (10) Applying Eq. (10) in Eq. (9), ′ Tiik = δℓmaknTℓmn = aknTmmn , (11) since the effect of multiplying by the Kronecker delta is to simply set ℓ = m in the remaining terms. If we define v T (where there is an implicit sum over i in T ), then, Eq. (11) yields k ≡ iik iik ′ vk = aknvn , which is the correct transformation law for a first-rank tensor, that is, a vector. Hence we have demonstrated that Tiik transforms like a vector. 5. Boas, problem 10.4–5. Given two point masses of equal mass m = 1 located at the coordinates (1 , 1 , 1) and ( 1 , 1 , 1), find the inertia tensor about the origin, and find the principal moments − and the principal axes. The moment of inertia tensor, with respect to the origin, for point masses is given by [cf. eq. (4.5) on p.506 of Boas and eq. (7.8) on p. 519 of Boas]: 2 I = m δ r x x , (12) ij a ij a − ai aj a X 2 where a labels the mass points, each of which is located at position (xa1,xa2,xa3), and r 2 2 2 ≡ x 1 + x 2 + x 3. Inserting the coordinates (1, 1, 1) and ( 1, 1, 1) into Eq. (12) yields the following a a a − matrix representation of Iij, 4 0 0 I = 0 4 2 . ij − 0 2 4 − The principal moments are the eigenvalues of Iij, which are determined by solving the character- istic equation, 4 λ 0 0 − 2 2 0 4 λ 2 = (4 λ) (4 λ) 4 = (4 λ) λ 8λ + 12 = (2 λ)(4 λ)(6 λ) = 0 . − − − − − − − − − − 0 2 4 λ − − 4 Thus, the principal moments are 2, 4 and 6. The principal axes correspond to the eigenvectors of Iij. Corresponding to λ = 2, 4 0 0 x x 0 4 2 y = 2 y , − 0 2 4 z z − which implies x = 0 and y = z. Corresponding to λ = 4, 4 0 0 x x 0 4 2 y = 4 y , − 0 2 4 z z − which implies y = z = 0. Corresponding to λ = 6, 4 0 0 x x 0 4 2 y = 6 y , − 0 2 4 z z − which implies x = 0 and y = z. Normalizing the eigenvectors, the above results yield the − following principal axes corresponding to λ = 2, 4 and 6, respectively, 0 1 0 1 1 1 , 0 , and 1 . √ √ 2 1 0 2 1 − 6. Show that a triple scalar product ~a ~b ~c is a pseudoscalar. Assume that ~a,~b and ~c are ordinary · × (i.e. polar) vectors. Hint: The transformation of the elements of Levi-Civita tensor is discussed in appendix D of the handout on tensors. We have ~a ~b ~c = ǫ a b c . (13) · × ijk i j k In appendix D it is shown that ǫ is a third-rank tensor under rotations. Since all the indices in Eq. (13) are contracted, we conclude that the triple scalar product is indeed a scalar under rotations.
Details
-
File Typepdf
-
Upload Time-
-
Content LanguagesEnglish
-
Upload UserAnonymous/Not logged-in
-
File Pages8 Page
-
File Size-