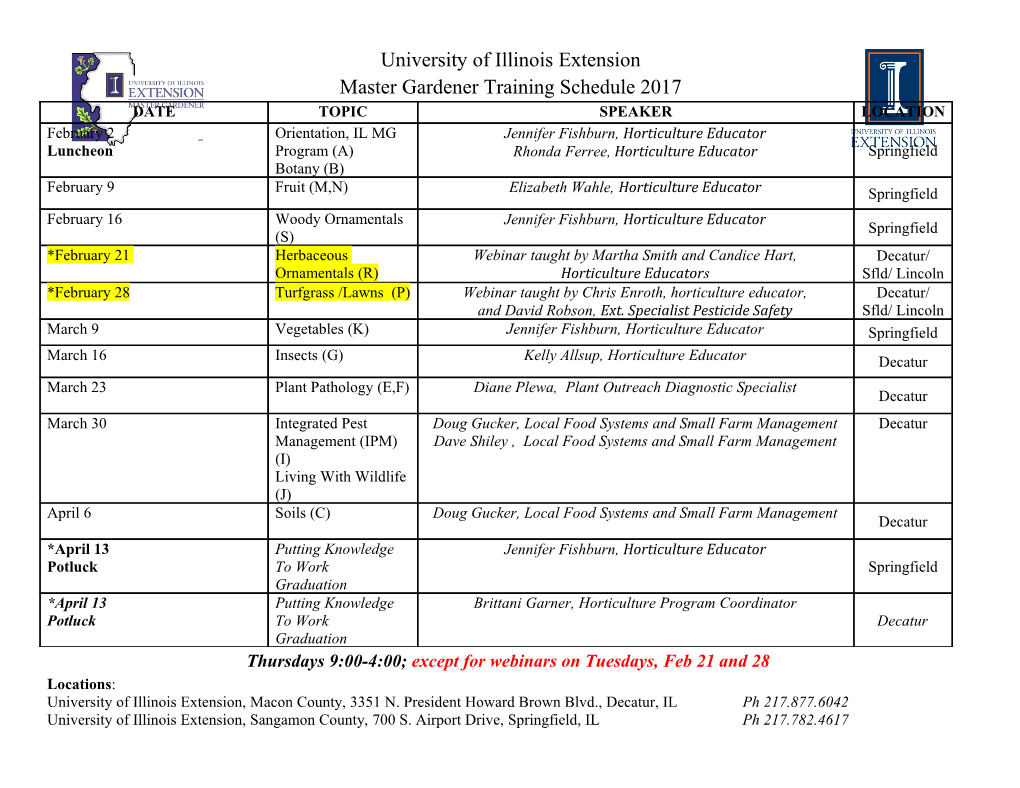
atoms Article Plasma Temperature and Electron Density Determination Using Laser-Induced Breakdown Spectroscopy (LIBS) in Earth’s and Mars’s Atmospheres Julian Stetzler, Shijun Tang and Rosemarie C. Chinni * Department of Math and Science, Alvernia University, Reading, PA 19607, USA; [email protected] (J.S.); [email protected] (S.T.) * Correspondence: [email protected]; Tel.: +1-610-568-1492 Received: 4 August 2020; Accepted: 21 August 2020; Published: 25 August 2020 Abstract: The purpose of this study was to calculate and compare the plasma temperatures and electron densities from the laser-induced breakdown spectroscopy (LIBS) data collected by NASA’s Martian rover and compare them to samples measured in Earth’s atmosphere. Using the Boltzmann plots, LIBS plasma temperatures were obtained for each site. The analysis focused on titanium lines that were located in the spectral region between 300 and 310 nm. The electron density was measured using the Stark broadening of the hydrogen line at 656.6 nm; the full width at half maximum (FWHM) of this line can be measured and correlated to the electron density of the plasma. Due to a neighboring carbon peak with the hydrogen line seen in many of the spectra from the Martian sites, the FWHM needed to be calculated using a computer program that completed the other side of the hydrogen line and then it calculated the FWHM for those data samples affected by this. The plasma temperatures and electron densities of the Martian sites were compared to LIBS samples taken on Earth. Keywords: atomic spectroscopy; LIBS; plasma temperature; electron density 1. Introduction The National Aeronautics and Space Administration (NASA) released over 300,000 data files from laser-induced breakdown spectroscopy (LIBS) studies conducted by the Mars Science Laboratory (MSL) Curiosity rover, which was launched in November of 2011. The ChemCam system on the Curiosity rover highlights many of the important applications of LIBS, and how LIBS is such a useful technique for analytical chemistry and planetary exploration. LIBS has been suggested as a useful technique for planetary exploration since 1990. The LIBS system aboard the MSL rover can provide elemental composition data on spots between 0.35 and 0.55 mm at distances over 7 m. Combined with the remote microimager (RMI), an image of the sample, and laser spot can aid in applying context to the data, while also operating at the same range. The remote-sensing capabilities are not the only reason the LIBS ChemCam system was an excellent choice for the Martian mission. The many applications of LIBS in detecting trace elements from carbon, oxygen, nitrogen, to heavy metals such as lead are invaluable for achieving the scientific objectives of the MSL. The scientific objectives of the curiosity rover were to explore the history of organic and volatile chemicals. The elements of interest, C, H, P, Mn, N, O, etc., can be detected remotely with LIBS. Additionally, the small spot size of the LIBS system allows it to target specific layers in the sedimentary rocks. This precise study of the sediment can be used to better understand the geological history of Mars and the history of carbon concentrations on Mars. The LIBS system could also be used to study hydration states and help understand the current water cycle on Mars. Finally, the LIBS system could be used to detect various toxic elements, such as lead, Atoms 2020, 8, 50; doi:10.3390/atoms8030050 www.mdpi.com/journal/atoms Atoms 2020, 8, 50 2 of 15 arsenic, etc., and determine their concentrations in the dust on Mars to better understand the hazards of the Martian environment for human exploration. This illustrates some of the many applications of LIBS to the Martian mission and planetary exploration but also shows some of the many important applications of LIBS as an analytical technique [1–3]. These many advantages of LIBS (i.e., the ability to work on any sample, remote-sensing capability, rapid analysis, and virtually no sample preparation) make it invaluable for the Martian mission. The key component of using LIBS as an analytical technique is the spectrum emitted by the plasma. The plasma formed from LIBS, which is used for any quantitative or qualitative analysis of a sample, has been studied since the 1960s. Important plasma characteristics such as the plasma temperature and electron density on different surfaces and under different conditions have been analyzed by multiple studies. However, the Martian mission offers a new and exciting opportunity, to study and compare LIBS plasmas formed on an entirely new celestial body to those formed on Earth. The environment of Mars, especially its atmospheric pressure and composition and other parameters could potentially affect the characteristics of the LIBS plasma. Therefore, analyzing the data gathered by the NASA rover could provide valuable insight on how environmental factors influence the plasma generated by LIBS, thus leading to a better understanding of LIBS plasma characteristics and using it for quantitative and qualitative analysis [4–7]. The formation of the plasma is an extremely complicated process, one that could be better understood. The formation of the plasma occurs via inverse bremsstrahlung (free electron interactions) and involves collisions between photons (particles of light), electrons and atoms/molecules. The expansion, transferring of energy throughout the surface, and the time at which the plasma decays are all dependent on factors such as the physical state of the sample, and whether it is in a vacuum. Meanwhile, the energy levels and excitation of atoms within the sample which form the plasma are dependent on the thermodynamic equilibrium and interactions which fall under the broad category of matrix effects [4]. Thermodynamic equilibrium is one that is not well understood. In many cases, it is approximated to be local thermodynamic equilibrium. The concept of thermodynamic equilibrium would allow the temperature of the plasma to describe the many different properties of plasma such as electron densities. The plasma characteristics are dependent on factors such as atmospheric composition and pressure, laser intensity and spot size. Additionally, electron densities measured at significantly different atmospheric pressures will produce results that are orders of magnitude different. Thus, it is expected that the electron densities on Mars and likely the plasma temperatures will be significantly different due to the significantly different atmospheric conditions on Mars [4,6]. However, it is also important to understand that the formation of the plasma itself, and the many ways to characterize it, are greatly affected by other factors. As previously mentioned, the surrounding atmospheric gases and pressure can affect the characteristics of the plasma such as the temperature and electron densities that are being studied. However, other factors such as the incident laser intensity, spot size and even the distance from the target surface can affect the plasma characteristics as well [1,3,8,9]. The Boltzmann plot method is a very common method for determining plasma temperatures. The Boltzmann plot method has proven to provide a precise average temperature of the plasma. It has proven more precise than alternatives such as the two-line Boltzmann method. Both methods compare the intensities of lines, but the two-line one compares the intensity ratios of only two lines to determine the plasma temperature, whereas the full Boltzmann plot does so graphically with multiple atomic emission lines [4,7]. Electron density is the number of free electrons, which are electrons separated from their atoms, per unit volume. Many studies use the well-established technique of Stark broadening to calculate electron densities, which has been shown to have good agreement between the experimental results and theoretical values. The line broadening in a LIBS plasma is mainly caused by Doppler width and the Stark effect. The Doppler width is dependent on the temperature and atomic mass of the emitting Atoms 2020, 8, 50 3 of 15 atoms. If only hydrogen lines are used for the electron density determination, the Doppler width can Atoms 2020, 8, x FOR PEER REVIEW 3 of 14 be disregarded, since it is only about 0.4–0.7 nm [8–10]. Theatoms. Stark If only eff ecthydrogen is a type lines of are pressure used for broadening. the electron density In a plasma, determination, this is attributedthe Doppler towidth interactions can causedbe bydisregarded, the collisions since ofit is primarily only about ions 0.4– but0.7 nm also [8– electrons.10]. The broadening of the hydrogen line is caused mostlyThe Stark by the effect Stark is a etypeffect. of As pressure mentioned broadening. previously, In a plasma, some of this the is broadening attributed to can interactions be attributed to thecaused Doppler by the width, collisions however, of primarily it is so minorions but that also it electrons. is disregarded. The broadening Therefore, of the hydrogen electron density line is can be determinedcaused mostly using by the Stark broadening effect. As of mentioned the Balmer-alpha previously, hydrogen some of the line broadening due to the can Stark be attrib effectuted [8 ,11]. Theto the purpose Dopplerof width, this studyhowever, was it tois so analyze minor athat subset it is disregarded. of the Martian Therefore, data for the electron electron densitydensity and can be determined using the broadening of the Balmer-alpha hydrogen line due to the Stark effect plasma temperature determination and then compare it to the data taken on Earth using similar laser [8,11]. energy parameters. The ChemCam data is publicly available [12]. The purpose of this study was to analyze a subset of the Martian data for electron density and plasma temperature determination and then compare it to the data taken on Earth using similar laser 2. Results energy parameters. The ChemCam data is publicly available [12].
Details
-
File Typepdf
-
Upload Time-
-
Content LanguagesEnglish
-
Upload UserAnonymous/Not logged-in
-
File Pages15 Page
-
File Size-