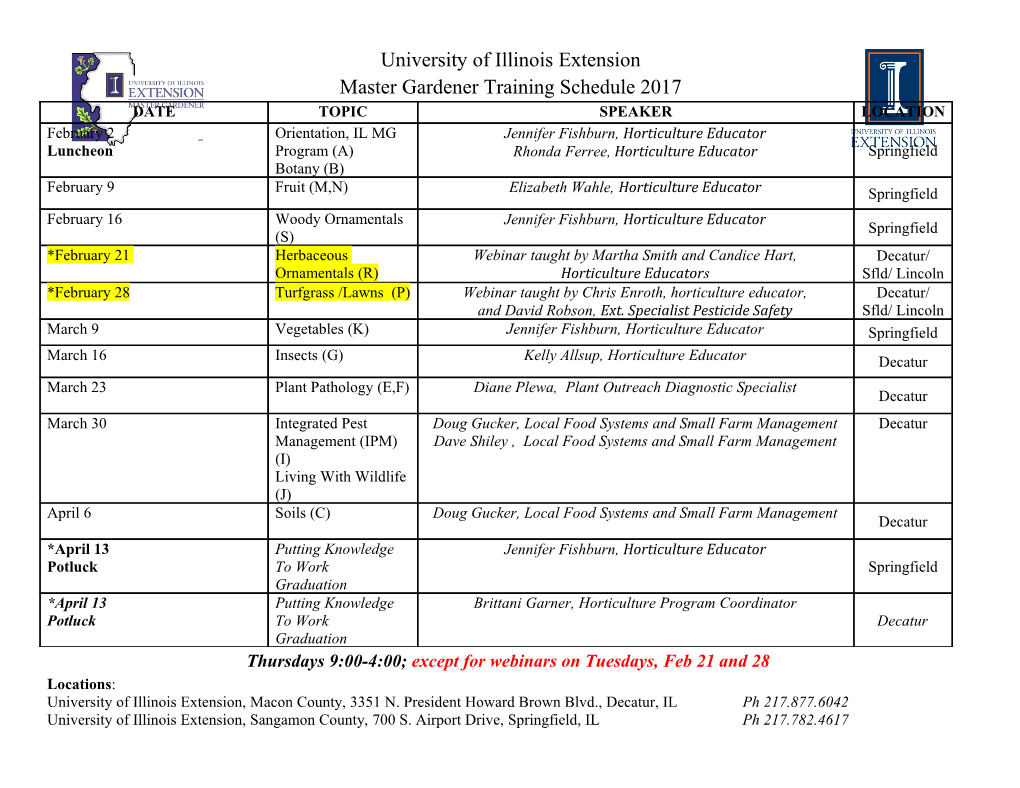
Bioastronomy 2007: Molecules, Microbes, and Extraterrestrial Life ASP Conference Series, Vol. 420, 2009 K. J. Meech, J. V. Keane, M. J. Mumma, J. L. Siefert, and D. J. Werthimer, eds. Methane Production on Rock and Soil Substrates by Methanogens: Implications for Life on Mars H. A. Kozup and T. A. Kral 1West Virginia University, WV 26505 2Arkansas Center for Space and Planetary Sciences, University of Arkansas, Fayetteville 72701 Abstract. In order to understand the methanogens as models for possible life on Mars, and some of the factors likely to be important in determining their abundance and distribution, we have measured their ability to produce methane on a few types of inorganic rock and soil substrates. Since organic ma- terials have not been detected in measurable quantities at the surface of Mars, there is no reason to believe that they would exist in the subsurface. Samples of three methanogens (Methanosarcina barkeri, Methanobacterium formicicum, and Methanothermobacter wolfeii) were placed on four substrates (sand, gravel, basalt, and a Mars soil simulant, JSC Mars-1) and methane production mea- sured. Glass beads were used as a control substrate. As in earlier experiments with JSC Mars-1 soil simulant, a crushed volcanic tephra, methane was pro- duced by all three methanogens when placed on the substrates, sand and gravel. None produced methane on basalt in these experiments, a mineral common in Martian soil. While these substrates do not represent the full range of materials likely to be present on the surface of Mars, the present results suggest that while some surface materials on Mars may not support this type of organism, others might. 1. Introduction The possibility of life on Mars has been a subject of intense interest for many decades, but only recently have we learned enough about Martian surface con- ditions to seriously address the issue. The Viking missions of the mid-1970s, though a spectacular engineering accomplishment, shed little light on the issue, yielding results that initially appeared to be positive, but were subsequently interpreted by most researchers as reflecting an unexpected inorganic chemistry. However, the Viking missions did confirm that conditions at the surface are rather harsh, conceivably too harsh to support any known life forms (Klein, 1978; Klein, 1979; Klein et al., 1992). The possibility of life below the surface is another matter. Recent evidence for global distributions of subsurface ice (Boynton et al., 2002; Feldman et al., 2002; Mitrofanov et al., 2002) and indica- tions that liquids flowed on the surface in recent times (Christensen et al., 2004; Herkenhoff et al., 2004; Klingelhofer et al., 2004; Rieder et al., 2004; Squyres et al., 2004a; Squyres et al., 2004b) mean that the possibility of extremophiles existing below the surface cannot be entirely excluded. We have been exploring the possibility that methanogens, microorganisms in the domain Archaea, could survive on Mars in subsurface environments. In general, methanogens metabo- 137 138 Kozup and Kral lize H2, using CO2 as carbon source, without a need for organic carbon (even though they are ubiquitous in environments associated with the decomposition of organic matter [Sowers, 1995]). The recent discovery of methane in the atmo- sphere of Mars is highly significant in connection with the possible presence of these organisms on Mars (Formisano et al., 2004; Malik, 2004; Krasnopolsky et al., 2004; Krasnopolsky, 2005). One factor that must be considered is whether there are reasonable soil/rock substrates – reasonable in terms of what we know about the surface of Mars – that would support methanogenesis on the planet. We have shown that three species of methanogens produce methane when in- cubated on the Mars soil simulant, JSC Mars-1 (Kral et al, 2004), a crushed volcanic tephra from Hawaii. However, as in all simulation experiments, there is an issue as to whether this substrate is relevant to Mars, and if so, how widespread such material might be. We have therefore proposed to examine the suitability of a few other inorganic substrates, in order to explore whether the identity of the substrate is important and, if so, gain some insight into the type of materials likely to support methanogens on Mars. Since organic materials have not been detected in measurable quantities at the surface of Mars (Bie- mann et al., 1977; Biemann, 1979), there is no reason to believe that they would exist in the subsurface. Here we report attempts to measure methanogenesis on a number of substrates, sand and gravel which are typical streambed deposits, basalt which might be expected in the vicinity of the huge volcanic regions on Mars, and a Mars soil simulant, JSC Mars-1. 2. Materials and Methods 2.1. Cultures and Growth Media The methanogens were obtained from David Boone, Portland State Univer- sity, OR. Each methanogenic strain was grown in a medium that supported growth (MS medium [Boone et al., 1989] for Methanosarcina barkeri [OCM 38] and Methanobacterium formicicum [OCM 55]; MM medium [Xun et al., 1988] for Methanothermobacter wolfeii [OCM 36; formerly Methanobacterium wolfei]. Growth media were prepared under 90% carbon dioxide and 10% hydrogen in a Coy anaerobic environmental chamber. Media made in the described atmo- sphere were saturated with carbon dioxide. This resulted in a pH of 6.6, which allows for good growth of the methanogens being studied. The 10% hydrogen is only required for the oxygen-removing palladium catalysts to function prop- erly. The catalysts facilitate the reaction of the residual molecular oxygen with the molecular hydrogen to form water. (Methanogens are strict anaerobes and will not grow or produce methane in the presence of molecular oxygen [Zin- der, 1993].) The anaerobically-prepared media were added to anaerobic culture tubes and sealed as described by Boone et al. (1989). At least one hour prior to inoculation, a sterile 2.5% sodium sulfide solution was added to each tube (0.15 mL per 10 mL medium) to eliminate any residual molecular oxygen (Boone et al., 1989). 2.2. Preparation of the Soil and Rock Samples Four different soil and rock samples were used in these experiments. They in- cluded a Mars soil simulant [JSC Mars-1], sand, gravel, and basalt. A fifth Methane Production 139 substrate, glass beads (Pyrex brand), served as a negative control. JSC Mars-1 is a fraction from altered volcanic ash from a Hawaiian cinder cone that approx- imates the reflectance spectrum, composition, grain size, density, and magnetic properties for the oxidized soil of Mars (Allen et al., 1998). The sand (silicate [SiO2] mineral [Leet, 1982]) and the gravel (natural mixture of various rock frag- ments resulting from erosion [Leet, 1982]) were from Quickrete Companies, Inc., Atlanta GA. The basalt (pulverized) was obtained from Todd Stevens, Portland State University. It is composed of plagioclase feldspars and ferromagnesium silicates (Leet, 1982). The sand and gravel were washed in deionized water to remove any loose de- bris and fine-grained dust. Because of the small grain size of the JSC Mars-1 and the basalt, washing was not feasible. Following the washing procedure, the sand, gravel and basalt were analyzed for total organic compounds at the Arkansas Wa- ter Resource Center, Water Quality Laboratory, University of Arkansas, Fayet- teville, using an Inductively Coupled Argon Plasma Spectrophotometer. (JSC Mars-1 had previously been tested for total organic compounds [Kral et al., 2004]). Five grams of each type of soil or rock were placed into anaerobic cul- ture tubes. All of the tubes containing soil and rock samples were placed into a Coy anaerobic chamber, allowed to acclimate to the hydrogen:carbon dioxide atmosphere for 24 hours, then stoppered. The sealed tubes were removed from the chamber, crimped, and autoclaved at 121C for 30 minutes at 100 kPa above ambient. 2.3. Methane Production on Different Soil and Rock Substrates Actively growing cells (approximately 0.1 O.D. at 675nm) of all three methan- ogens were centrifuged at 4200 rpm for 45 min, and then washed with sterile carbonate buffer (the same buffer used to make methanogenic growth medium). At least an hour earlier, sterile sodium sulfide solution was added to the buffer to eliminate residual oxygen. This washing procedure was repeated three times. Following the final washing, the cell pellets were suspended in the same buffer. Four milliliters of each organism were added to individual tubes of each of the soil and rock substrates. (We have shown previously [Kral et al., 2004] that 4 mL is ideal for growth on 5 g of JSC Mars-1.) All tubes were pressurized with 180 kPa (above ambient) of 75:25 H2:CO2. The tubes were incubated at temperatures within the growth range for the respective methanogens (37 oC for M. barkeri and M. formicicum; 55 oC for M. wolfeii). Methane was measured as a percentage of the total headspace gas. Headspace gas samples (1 mL) were removed with a plastic syringe at time intervals of 0, 72, 144, 312 and 480 hours after inoculation and manually injected into the port of a Hewlett Packard model 5890 gas chromatograph with a thermal conductivity detector at an oven temperature of 40 oC using argon as the carrier gas. 2.4. Transfer Experiments Washed cell cultures mixed with soil and rock samples were prepared as de- scribed in the previous section. Gas chromatographic measurements were record- ed at 0, 72, and 144 hours. At 168 hours after inoculation, 4 mL of sterile methanogenic medium buffer (with sodium sulfide) were added to each culture tube in the anaerobic chamber using a sterile syringe. The tubes were inverted 140 Kozup and Kral a few times and then allowed to settle for five minutes. The tubes were then carefully inverted, one at a time, allowing the liquid fraction to collect at the stopper end of each tube.
Details
-
File Typepdf
-
Upload Time-
-
Content LanguagesEnglish
-
Upload UserAnonymous/Not logged-in
-
File Pages10 Page
-
File Size-