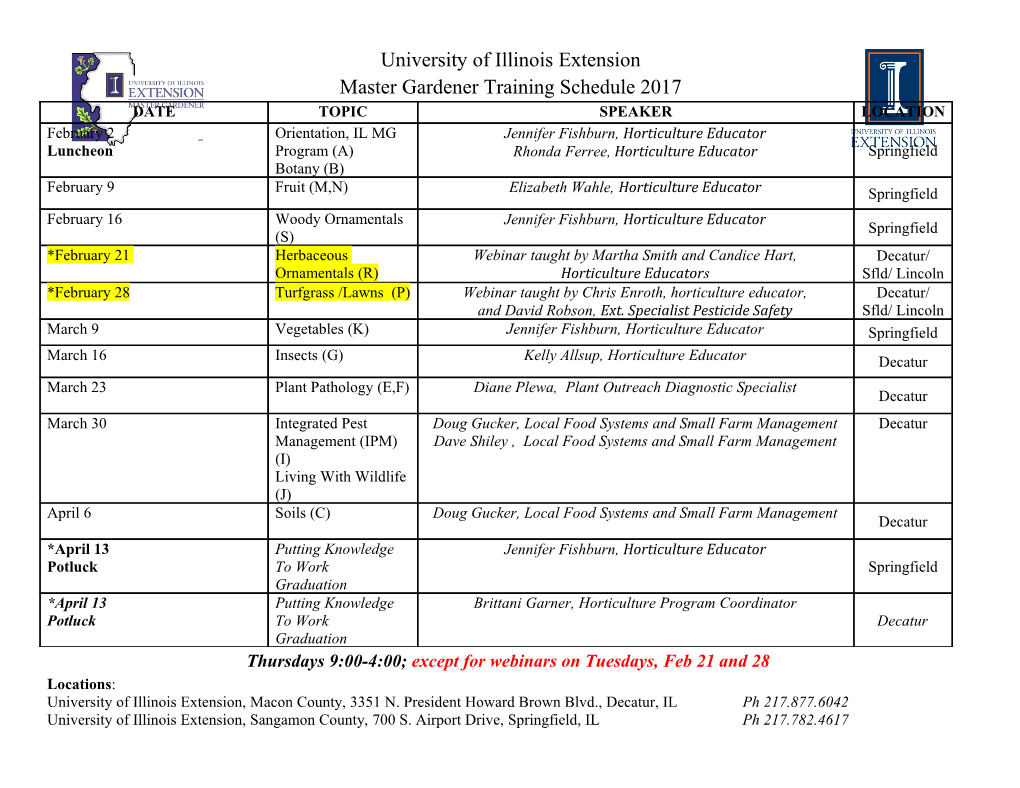
Proton Colliders: Tevatron and LHC Why build bigger better accelerators? Why proton colliders? Fermilab’s Tevatron CERN’s LHC 1 The Standard Model describes: Matter... …and the forces that act on matter. Force: carrier: acts on: 2 However, the Standard Model does not explain everything, e.g.: • Why do particles have mass? • Why three generations of quarks and leptons? • Are quarks and leptons really fundamental? • Why the matter-antimatter asymmetry? • What is the "dark matter" in the cosmos? • How does gravity fit in? 3 The Higgs Boson • The Higgs Mechanism provides a way to give particles mass, but requires the existence of a particle - as yet unobserved - called the Higgs Boson. • Current (experimental) lower bound on the Higgs Boson mass is ~110 GeV • Upper bound on Higgs mass (set by theory) is ~1 TeV 4 Extensions to the Standard Model • Numerous theories exist to try to answer questions that the Standard Model leaves open. • Some of these theories predict the existence of other new particles at an energy scale of ~ 1 TeV. 5 Motivation for New Accelerators • Look for new particles • Study properties of known particles more and more precisely as any deviation from Standard Model predictions would signal new physics. 6 Tevatron and LHC • The world’s most powerful accelerator is the Tevatron at the Fermi National Accelerator Laboratory near Chicago. – Proton-antiproton (‘p-pbar’) collider – centre-of-mass energy @ 2 TeV – running since 1987 • A new accelerator, called the Large Hadron Collider (LHC) is being constructed at CERN near Geneva. – Proton-proton (‘p-p’)collider – centre-of-mass energy @ 14 TeV – start-up in ~2006 7 Key Properties of Accelerators • The type of particle to be accelerated • The energy to which the particles will be accelerated • Luminosity • The fraction of the beam energy that is useful for creating new particles 8 What type of particle to accelerate? • Particles must be – charged: • accelerated by electric fields • steered and focussed using magnetic fields – Long-lived which limits us to protons and electrons. • How to choose…? 9 Beam Energy • For high-energy beams, must do one of the following: – pass the particles through very large electric fields • Þ technological limitations... – pass them through many smaller electric fields placed in a line • Þ need many cavities and a long line... – pass them through the same smaller electric field many times • Þ circular path via dipole magnets placed around ring: p(TeV)=0.3 B(Tesla) * R(km) • For the last option: delicate balancing act required: particle energy constantly increasing, must increase electric and magnetic fields synchronously Þ ‘synchrotron’. 10 Synchrotron Radiation • As charged particles travel in a curved orbit, they lose energy; per-revolution energy loss is 2 3 4 DE = q b g / 3e0R • Note that since g=E/m, electrons lose energy about 1013 times faster than protons! • LEP was ~ at practical energy limit for e+e- – energy lost to synchrotron radiation had to be continuously replaced 11 Luminosity In circular accelerators, particles travel in bunches. Luminosity L = f n k2 / A (cm-2 sec-1) where: • f = the frequency of circulation • n = the number of bunches around the ring • k = the number of particles per bunch • A = the cross-sectional area of the bunch 12 Luminosity and Cross-Section • The number of events of a particular type in time T is N = s L T where s = the cross-section for the type of event (i.e. s is a measure of how likely it is that the process will occur.) • A note about units: – luminosity is measured in cm-2 sec-1 – cross-sections are measured in ‘barns’; 1 ‘barn’ = 10-28 m2 – Amounts of data (‘integrated luminosities’) are often measured in ‘inverse barns’ • e.g. in a good day, LEP could produce 3 pb-1 of data 13 p-p(bar) Luminosities and Cross-sections • Interesting events are rare (i.e. have very low cross- sections), so • Need L to be as high as possible to maximise number of interesting events available. 14 How much beam energy is really available for making new particles? In an e+e- collider: all of it. In a p-p(bar) collider: not so simple... 15 Phenomenology of p-p(bar) collisions Most p-p(bar) collisions are ‘soft’, i.e. due to large-distance collisions between the two incoming protons: – momentum transfer is small. – most of the final-state particles’ momentum is longitudinal (parallel to beam direction); p^ (transverse momentum) is small. – These are called ‘minimum bias’ events. – They are not interesting. 16 Phenomenology of p-p(bar) collisions, continued... The interesting events are due to ‘hard scattering’: small-distance interactions between two partons (constituent quarks and gluons) of the incoming protons: – momentum transfer is large so • massive particles can be created • final-state particles can have large p^ – very rare compared to minimum bias 17 A typical LEP event In an e+e- collision, leptons annihilate completely Þ e+e- events are very ‘clean’ ; interesting final- state particles only. 18 A typical LHC event Ü An event in an LHC detector: most tracks are due to minimum bias Ü The same event after requiring p^ > 2 GeV. 19 How massive can the created particles be? In p-p(bar) hard scattering, partons annihilate (not the whole proton) so the effective centre-of-mass energy is smaller than energy of the machine: ÖS = Ö(xaxbs) where xa and xb are the fractions of the proton momentum carried by the two colliding partons 20 What are these ‘colliding partons’? • Proton is made of many partons: – three valence quarks (uud), – and the sea: a colourless collection of quarks, anti-quarks and gluons. • At low momentum transfer (Q2): – sea partons carry little momentum – valence quarks share ~all proton momentum Þ xi distribution peaks at ~1/3 • At higher Q2: – sea begins to carry more momentum – xi spectra of valence quarks are shifted down – still possible to have xi @ 1/3, but less likely • A collision between high-energy beams can achieve higher Q2 than a collision between low-energy beams. 21 OK - So how massive can the created particles be? • Recall the effective centre-of-mass energy ÖS = Ö(xaxbs) • But xa and xb are probability distributions, not hard numbers… • …so there is no clear answer. 22 Fermilab’s Tevatron – Located near Chicago, Illinois (USA) – first p-pbar collisions in 1986 with: • Ös = 1.8 TeV • L = 1030 cm-2 sec-1 – Currently: • Ös @ 2 TeV • L @ 1032 cm-2 sec-1 – 1995: first observation of top quark ! 23 The Fermilab Chain of Accelerators – Cockcroft-Walton pre-accelerator: H Þ H- Þ 750 keV – linear accelerator: H- Þ 400 MeV Þ C foil Þ protons – Booster: protons Þ 8 GeV – Main Injector: protons Þ 120 GeV Þ pbar production p,pbar Þ 150 GeV – Tevatron: p,pbar Þ 1 TeV Þ collisions ! – Antiproton Recycler: takes pbar from Tevatron to use again 24 Tevatron Experiments: • CDF and DZero: general-purpose detectors – searches for new particles – precision measurements – first observers of top quark (1995) 25 CERN Large Hadron Collider (LHC) • being installed in LEP tunnel (27 km circumference) • to start operation in 2006 • p-p and heavy ion (e.g. Pb-Pb) collisions 26 The LHC in pp mode: – centre-of-mass energy (Ös) = 14 TeV – luminosity: • first few years: luminosity = 1033 cm-2 s-1 • design luminosity = 1034 cm-2 s-1 • TeV and LEP : ~1032 cm-2 s-1 – can search for new particles with masses in the TeV range 27 The LHC Proton Injection Chain – Linear accelerator: • protons Þ 50 MeV – Proton Synchrotron (PS): • 50 MeV Þ 26 GeV • Super Proton Synchrotron (SPS): • 26 GeV Þ 450 GeV • NB itself once the world’s most powerful synchrotron. W ±, Zo discovered here in early 1980’s. • LHC • 450 GeV Þ 7 TeV Þ collisions ! 28 LHC Experiments: – ATLAS and CMS • general-purpose pp experiments • searches for new particles • precision measurements – LHCb • b-hadron physics • CP violation – ALICE • study heavy-ion collisions 29 Why is LHC p-p, not p-pbar? • A p-pbar collider is mechanically simpler than a p-p collider: – beams have equal-and-opposite charge, so can use same beampipe, same magnets, same RF cavities, etc. • p-p is more complicated: – need 2 separate beampipes – need either a separate set of RF cavities and magnets, or very complicated ones – beam-crossing is trickier 30 p-p vs. p-pbar, continued • However: antiprotons are difficult to make. • Tevatron’s antiproton source: – 120 GeV protons collide with Nickel target – many secondary particles produced, including antiprotons, but only about 1-2 antiprotons per 105 protons! – Must then sort out the antiprotons from all the rest of the secondary particles... 31 p-p vs. p-pbar, p. 3 Also - remember xi? – at low Q2: • valence quarks carry most of the proton’s momentum: • p-pbar @ quarks-on-antiquarks; more likely to annihilate than p-p (@ quarks-on-quarks) – at high Q2: • sea quarks and antiquarks become more ‘visible’: • p-pbar loses some of its advantage over p-p 32 LHC is p-p, not p-pbar, because: • Luminosity must be as high as possible • The easiest way to boost luminosity is to increase the number of particles per bunch, but – antiprotons are hard to make... – ...and anyway as energy increases p-p cross-sections begin to look like p-pbar cross-sections. P-p starts looking better than p-pbar for very high energies. 33.
Details
-
File Typepdf
-
Upload Time-
-
Content LanguagesEnglish
-
Upload UserAnonymous/Not logged-in
-
File Pages33 Page
-
File Size-