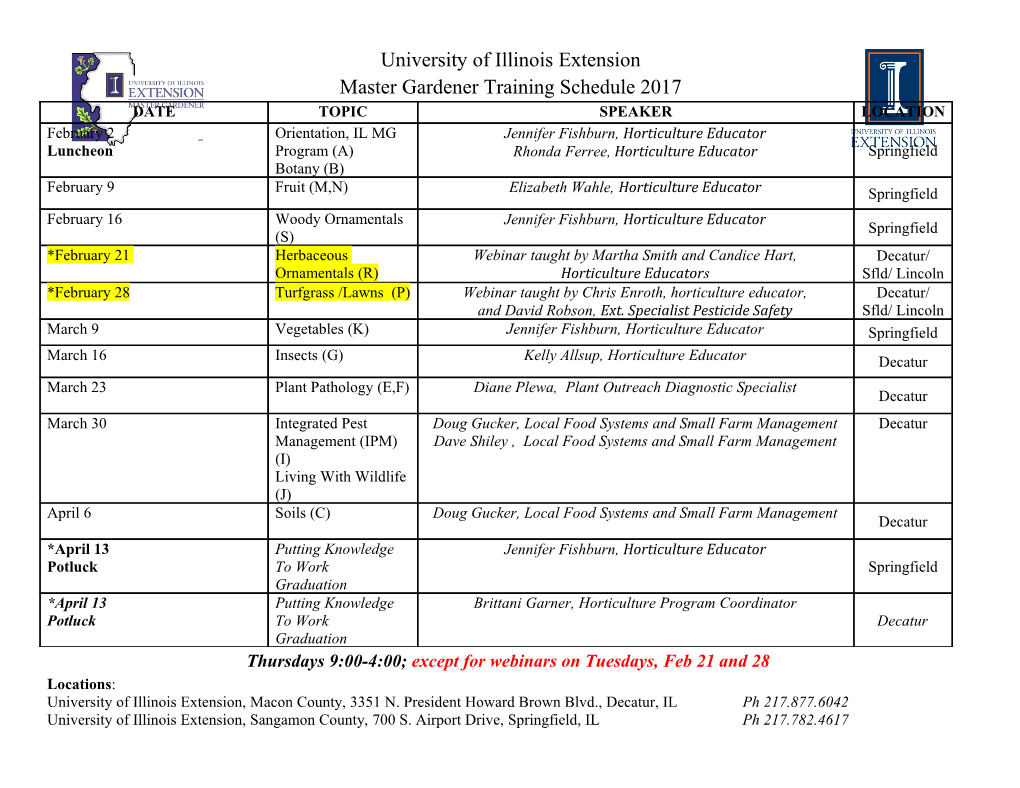
Serine and threonine are often phosphorylated in proteins that participate in cell-cell communication. This modification is reversible and serves as a chemical, signal transduction tag. The two amino acids may also function as nucleophiles in enzymatic catalysis, thanks to the hydroxyl group in their side chain. Serine and threonine on the surface of membrane/secreted proteins may also be glycosylated (attached with sugar groups). This protects the protein and increases its water solubility. In some cases, the attached sugar groups serve also as a recognition code for other extra-cellular elements. Cysteine, despite being polar, tends to appear in core of water-soluble proteins. Under oxidizing conditions, its thiol side chain deprotonates and tends to form a (covalent) disulfide bond with a thiol group of a neighboring cysteine. These bonds are important for stabilizing proteins that are secreted from cells (hormones, antibodies, some enzymes). Cysteine also serves as anucleophile and an electron-transfer agent in enzymatic catalysis. Both are the result of the non-bonding electrons of cysteine’s sulfur atom, conferring it high chemical reactivity and the ability to exist in different oxidation states. The carboxamide group of glutamine and asparagine can serve as both hydrogen bond donor and acceptor. As a result, these amino acids are commonly involved in hydrogen bond networks within proteins. This group in asparagine is also glycosylated in membrane/secreted proteins (see above for the benfits of glycosylation). d III. Polar-charged amino acids These include the following (Fig. 4): Aspartate (asp, D) and glutamate (glu, E) – have a carboxyl group in their side chains. As explained above, this group has a low pKa and therefore tends to become deprotonated and negatively charged at physiological pH. For this reason, aspartate and glutamate are referred to as ‘acidic‘. In proteins, they tend to interact electrostatically with positively charged groups in other amino acids or in the protein’s ligand/substrate. Lysine (lys, K) and arginine (arg, R) – have nitrogen-containing groups in their side chains (amino group in lysine and guanidine group in arginine). These groups have high pKa and therefore tend to become protonated and positively charged at physiological pH. For this reason, lysine and arginine are referred to as ‘basic‘. In proteins, they tend to interact electrostatically with negatively charged groups in other amino acids or in the protein’s ligand/substrate. Histidine (his, H) – has an imidazole group in its side chain. This group has a pKa of ~6, and therefore has a 50% chance of being protonated (positively charged) or deprotonated (neutral) at physiological pH. This allows histidine to function in hydrogen-transfer enzymatic catalysis, where it may functions as the hydrogen donor, acceptor, or both. Here are interesting facts on some polar-uncharged amino acids: Carboxylation of glutamate on its γ carbon (see Fig. 6 below) allows it to bind efficiently cations, such as divalent calcium. This is very useful in clotting proteins, such as prothrombin, the activity of which is regulated by blood calcium levels. The amino group of lysine’s side chain is able to form Schiff base with aldehydes. This helps some proteins bind aldehyde-containing prosthetic groups (e.g. the pyridoxal phosphate coenzyme of aminotranferase enzyme). Oxidation of lysine’s side chain in structural proteins like collagen allows it to participate in cross- linking reactions that stabilize these proteins. As explained above, the imidazole side chain of histidine can serve as both acid and base thanks to its pKa, which is close to the physiological pH. This is important, for example, for the catalytic mechanism of the enzyme acetylcholine esterase, which inactivates the neurotransmitter acetylcholine. The inactivation is important for preventing our nervous system from going into paralysis and death, which is what happens when the enzyme is attacked and inactivated by toxic nerve agents. Acetylcholine is an ester, and it is hydrolyzed to choline and acetate by the enzyme, via nucleophilic attack of a catalytic serine residue on the ester bond. To do that, the serine must deprotonate, and this is made possible by a nearby histidine which acts as a base, abstracting serine’s proton: IV. Aromatic amino acids These includes the following (Fig. 5): Phenylalanine (phe, F) – has a phenyl group in its side chain. Tyrosine (tyr, Y) – has a phenol group in its side chain. Tryptophan (trp, W) – has an indole group in its side chain In contrast to the other amino acids groups, this one is considered not according the polarity of the side chain but rather on its aromatic nature (in the chemical sense, not the olfactory one…). Aromatic groups contain delocalized π electrons which can interact with similar electrons in other aromatic groups, as well as with positively-charged groups. Indeed, all of these interactions are observed in proteins between aromatic side chains. The aromatic amino acids are important in forming closed scaffolds within proteins, especially binding sites for ligands and substrates. Here are interesting facts on some aromatic amino acids: Like serine and threonine, tyrosine may also become phosphorylated on proteins involved in cellular communications. A well-known example is the membrane-bound receptors which respond to growth factors. Binding of the latter to these receptors results in their phosphorylation, which in turn conveys the signal into the cell and results in cellular division. Genetic defects that allows for hormone- independent phosphoryaltion of these proteins often lead to cancer. The phenol group of tyrosine also participates in differnt mechanisms of enzymatic catalysis (e.g. nucleophilic attack, acid-base catalysis and stabilization of reaction intermediates). The indole group in tryptophan’s side chain is capable of participating in different polar and nonpolar non-covalent interactions with other chemical fspecies. Therefore, it is common in protein binding sites. It also participates inenzymatic catalysis and electron transfer. Tryptophan’s side chain fluoresce when absorbing UV light. This allows biochemists to identify proteins or study changes in their structure by UV-irradiating them and then recording the fluorescence of their tryptophan amino acids. Selenocysteine and pyrrolysine Selenocysteine and pyrrolysine are two amino acids derivatives that evolution found a way to genetically incorporate into certain proteins. For this reason they are often referred to as the ’21st & 22nd amino acids’. Protein incorporation is achieved by loading these amino acids on a specialized tRNA molecule, which binds inside the ribosome to a mRNA ‘stop’ codon (UGA in the case of selenocysteine and UAG in the case of pyrrolysine). A stop codon normally signals the translation machinery to stop translation, but in the case of selenocysteine and pyrrolysine the codon also contains an addition sequence motif which prevents termination. Selenocysteine is very similar to cysteine, with the sulfur atom replaced by selenium. However, it is created enzymatically from serine rather than from cysteine. The replacement of sulfur with selenium turns (seleno)cysteine into a better nucleophile, which is an advantage in enzymatic catalysis. Indeed, selenocysteine appears in certain oxidation-reduction enzymes. Some selenocysteine-containing enzymes, like glutathione peroxidase and some forms of thioredoxin reductase, act as antioxidants. That is, they are part of the body’s built-in system that fights and reverses oxidative damage, caused by free radicals. In addition, selenocysteine appears in enzymes that form T3, the most potent thyroid hormone. This is why selenium in the diet is important for the function of the thyroid gland. Pyrrolysine appears in an enzyme (methyltransferase) of methane-producing Archaea and 0ne Eubacterium. It is enzymatically created from the natural amino acid lysine. dd II. Amino acids that are formed post-translationally In some cases, a natural amino acid already present in a protein can be enzymatically modified to form a chemical derivative. For example, collagen, a protein that is widespread in animal connective tissues, contains hydroxyproline and hydroxylysine. These are produced by attachment of a hydroxyl group to the natural amino acids proline and lysine, respectively. Another non-natural amino acid, γ-carboxyglutamate, is form ed by attachment of a carboxyl group to the amino acid glutamate in blood-clotting proteins. Figure 6a. Some examples of non-natural amino acids. ff III.D-amino acids Amino acids with the opposite configuration (D-amino acids), can be found in some proteins and peptides. Most of the latter are bacterial proteins/peptides. For example, the bacterial cell wall (peptidoglycan) contains D- alanine, while the bacterial antibiotic valinomycin contains D-valine. However, D-amino acids can also be found in multicellular organisms. For example, a peptide called dermorphin, which is produced by a South American frog to repel predators, contains D-alanine. Figure 6b. Two peptides containing D-amino acids. sd d 5. AMINO ACIDS AS A SOURCE OF OTHER COMPOUNDS Some amino acids, such as tyrosine, tryptophan, histidine and glutamate serve as precursors of ‘biogenic amines‘, compounds that are non-proteogenic (do not appear in proteins), yet have different cellular & physiological roles. Many biogenic amines act as neurotransmitters, hormones, or local mediators. Here are some
Details
-
File Typepdf
-
Upload Time-
-
Content LanguagesEnglish
-
Upload UserAnonymous/Not logged-in
-
File Pages11 Page
-
File Size-