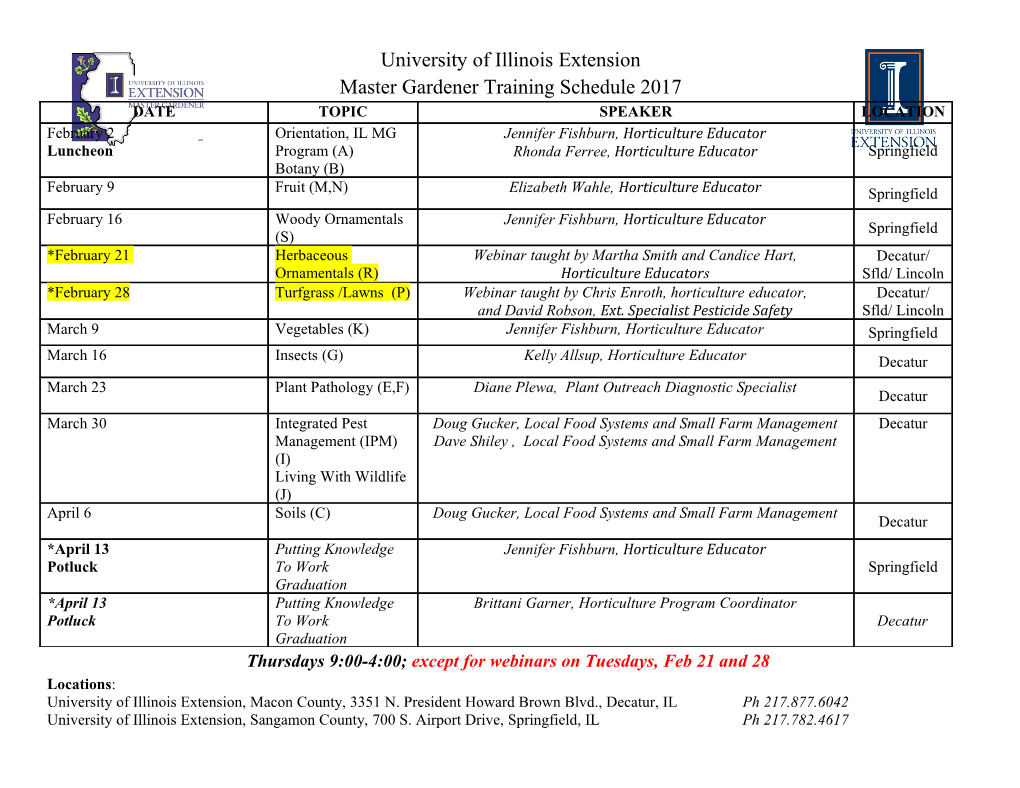
ORIGINAL RESEARCH published: 27 October 2016 doi: 10.3389/fphys.2016.00482 The Bohr Effect Is Not a Likely Promoter of Renal Preglomerular Oxygen Shunting Ufuk Olgac 1, 2 and Vartan Kurtcuoglu 1, 2, 3* 1 The Interface Group, Institute of Physiology, University of Zurich, Zurich, Switzerland, 2 National Center of Competence in Research, Kidney.CH, Zurich, Switzerland, 3 Zurich Center for Integrative Human Physiology, University of Zurich, Zurich, Switzerland The aim of this study was to evaluate whether possible preglomerular arterial-to-venous oxygen shunting is affected by the interaction between renal preglomerular carbon dioxide and oxygen transport. We hypothesized that a reverse (venous-to-arterial) shunting of carbon dioxide will increase partial pressure of carbon dioxide and decrease pH in the arteries and thereby lead to increased oxygen offloading and consequent oxygen shunting. To test this hypothesis, we employed a segment-wise three-dimensional computational model of coupled renal oxygen and carbon dioxide transport, wherein coupling is achieved by shifting the oxygen-hemoglobin dissociation curve in dependence of local changes in partial pressure of carbon dioxide and pH. Edited by: The model suggests that primarily due to the high buffering capacity of blood, there is Timothy W. Secomb, University of Arizona, USA only marginally increased acidity in the preglomerular vasculature compared to systemic Reviewed by: arterial blood caused by carbon dioxide shunting. Furthermore, effects of carbon Daniel Goldman, dioxide transport do not promote but rather impair preglomerular oxygen shunting, University of Western Ontario, Canada as the increase in acidity is higher in the veins compared to that in the arteries. We Brendan Fry, Metropolitan State University of conclude that while substantial arterial-to-venous oxygen shunting might take place in Denver, USA the postglomerular vasculature, the net amount of oxygen shunted at the preglomerular *Correspondence: vasculature appears to be marginal. Vartan Kurtcuoglu [email protected] Keywords: renal oxygenation, oxygen shunting, carbon dioxide shunting, pH, Bohr effect Specialty section: This article was submitted to INTRODUCTION Computational Physiology and Medicine, Hypoxic conditions in the renal cortex or in the medulla may induce tissue damage and contribute a section of the journal to the pathogenesis of acute and chronic kidney diseases (Evans et al., 2008). It is therefore essential Frontiers in Physiology to understand the mechanisms that are involved in the regulation of renal oxygenation. We have Received: 13 July 2016 shown previously that preglomerular arterial-to-venous (AV) oxygen shunting—a mechanism Accepted: 07 October 2016 hypothesized to contribute to the regulation of renal oxygenation (Leong et al., 2007; Evans et al., Published: 27 October 2016 2008)—is unlikely to be significant (Olgac and Kurtcuoglu, 2015a,b). Since then the question Citation: has arisen whether substantial shunting may nevertheless exist, enabled by the influence of Olgac U and Kurtcuoglu V (2016) The Bohr Effect Is Not a Likely Promoter of preglomerular carbon dioxide dynamics on oxygen transport. Here we hypothesize that reverse, Renal Preglomerular Oxygen venous-to-arterial (VA) shunting of carbon dioxide will increase arterial partial pressure of carbon Shunting. Front. Physiol. 7:482. dioxide, decrease pH, and thereby lead to augmented oxygen offloading through the Bohr effect doi: 10.3389/fphys.2016.00482 (Kilmartin and Rossi-Bernardi, 1973; Dash et al., 2016) and consequent AV oxygen shunting. Frontiers in Physiology | www.frontiersin.org 1 October 2016 | Volume 7 | Article 482 Olgac and Kurtcuoglu Bohr Effect and Oxygen Shunting Measurements of renal carbon dioxide partial pressure (PCO2 ) vessels and the tissue. The vessels are composed of a RBC-rich and pH indicate a wide range of values in various structures of region in the core and a RBC-free region close to the walls. The the kidney: In the proximal tubule, PCO2 was measured by Sohtell thickness of the RBC-free region in vessels of each Strahler order (1979) to be 60.6 mmHg, by DuBose et al. (1979) 65 mmHg, 2 2 is given by δ = R + tRBC − R − R , where R is the vessel by Maddox et al. (1984) 57.1–62.1mmHg, and by De Mello RBC radius in that order, and R and t are the radius (4 µm) Aires et al. (1990) 35.5 mmHg. In stellate vessels, reported values RBC RBC and maximum half thickness (1.3 µm) of a RBC, respectively are 33.7 mmHg (Sohtell, 1979), 65 mmHg (DuBose et al., 1979), (Nair et al., 1989). The plasma and RBC velocity profiles and the 57.1 mmHg (Maddox et al., 1984), and 38.9mmHg (De Mello hematocrit profile in the RBC-rich region, u (r), u (r) and Aires et al., 1990). pH of 6.7–7.06 was measured by DuBose et al. P RBC h (r), respectively, as well as the plasma velocity profile in the (1979) in the proximal tubule and of 7.27 in the stellate vessels. ′ ( ) These values suggest that, depending on the location within the RBC-free region, uP r , are calculated as explained in Olgac and Kurtcuoglu (2015a) and set on the computational domain prior renal cortex, PCO and pH may vary. However, the extent of the 2 to solving the governing equations for oxygen and carbon dioxide deviation of PCO2 and pH from the values of systemic arterial blood is not well known. Similarly, reliable quantitative values transport. of in vivo P and pH values inside red blood cells (RBCs) CO2 Oxygen Transport traversing the preglomerular vasculature are, to our knowledge, For the oxygen transport, we base our calculations on a derivative not obtainable experimentally. Since these data are necessary of our model presented in Olgac and Kurtcuoglu (2015a) where to test our hypothesis, a mathematical modeling approach is now the variability of P , i.e., the half saturation oxygen partial warranted. 50 pressure, is taken into account. Briefly, in the vessels, blood Renal CO trapping through a reverse shunting mechanism 2 plasma, and RBCs are axially convected. The plasma carries was previously hypothesized by Bidani et al. (1984) to occur in dissolved oxygen and the RBCs carry dissolved and hemoglobin- the postglomerular vasculature and by Atherton et al. (1988) bound oxygen. Radial diffusion of dissolved oxygen in the plasma and Schurek et al. (1990) in the interlobular artery-vein pairs is also accounted for. For a variable P , in the RBC-rich region, of the preglomerular vasculature. Schurek et al. (1990) further 50 P is governed by hypothesized that such a mechanism enhances AV oxygen O2 shunting through the Bohr effect. Here we tested this hypothesis P RBC CHbT dSO2 incorporating the latest structural data by Ngo et al. (2014) α 1 − h (r) uP (r) + α h (r) uRBC (r) 1 + RBC O2 O2 α dPO2 that account for the wrapping of veins around arteries in the O2 ∂P P dS preglomerular vasculature. To this end, we extended our previous O2 − O2 O2 ∂P50 ∂z h (r) uRBC (r) CHbT P dP ∂z computational model on renal oxygen transport (Olgac and 50 O2 P P α D ∂P Kurtcuoglu, 2015a,b) to include carbon dioxide transport. We = O2 O2 ∂ O2 r ∂r r ∂r , (1) then coupled oxygen transport to carbon dioxide transport through a function describing the shift of the oxygen-hemoglobin P RBC where CHbT, α and α are the total heme group dissociation curve with respect to the local PCO2 and pH O2 O2 as recently described by Dash et al. (2016). The resulting concentration in RBCs and the solubility of oxygen in plasma and inside the RBC, respectively (see Data Sheet 1 in Supplementary computational model is capable of quantifying PO , PCO and 2 2 Material for derivation). The permeability of oxygen in plasma is pH as well as fluxes of O2 and CO2 through the vessel walls of defined as KP = αP DP , where DP is the diffusion coefficient wrapped and not-wrapped artery-vein pairs in the preglomerular O2 O2 O2 O2 vasculature. Computations of oxygen transport were performed of oxygen in plasma. Note that the second term on the left hand side of Equation 1 vanishes for constant P , recovering the with a Hill equation with constant P50 as well as a variable P50 in 50 original equation developed in Olgac and Kurtcuoglu (2015a). In dependence of the local PCO and pH. 2 the RBC-free region, plasma free oxygen concentration follows: METHODS αP DP ′ ∂PO2 O2 O2 ∂ ∂PO2 αPu (r) = r . (2) Model Domain P ∂z r ∂r ∂r The three-dimensional (3D) model domain was previously described in Olgac and Kurtcuoglu (2015a,b). It consists of The tissue, together with its capillary vessels, is considered a representative levels corresponding to 11 Strahler orders of homogeneous structure in which perfusion and consumption the preglomerular vasculature. Each level contains wrapped are uniform and dependent on the fractional capillary volume, and not-wrapped artery-vein pairs. The structural information ϕ. Both diffusion of free oxygen and advection of free and for each level (artery radius, Ra, vein radius, Rv, number of hemoglobin-bound oxygen along the capillaries are taken into wrapped and not-wrapped vessels, kw and knw, respectively, account. The homogeneous tissue oxygen partial pressure, PO2 , lumen separation for wrapped and not-wrapped vessels, LSw and is governed by Salathe (1982) and Olgac and Kurtcuoglu (2015a): LSnw, respectively, and length of vessels, l) is given in Table 1. H C dS ϕuα ∇P 1 + c HbT O2 = αT DT ∇2P Mathematical Formulation T O2 T O2 O2 O2 α dPO Two different sets of equations governing oxygen and carbon O2 2 ˙ ˙ dioxide transport dynamics in the renal cortex are solved in the + (1−ϕ) (MO2 +Mc,O2 ),(3) Frontiers in Physiology | www.frontiersin.org 2 October 2016 | Volume 7 | Article 482 Olgac and Kurtcuoglu Bohr Effect and Oxygen Shunting where u, αT , H , M˙ and M˙ are the advection velocity of pH = 7.24 and P = 40 mmHg (Dash et al., 2016).
Details
-
File Typepdf
-
Upload Time-
-
Content LanguagesEnglish
-
Upload UserAnonymous/Not logged-in
-
File Pages14 Page
-
File Size-