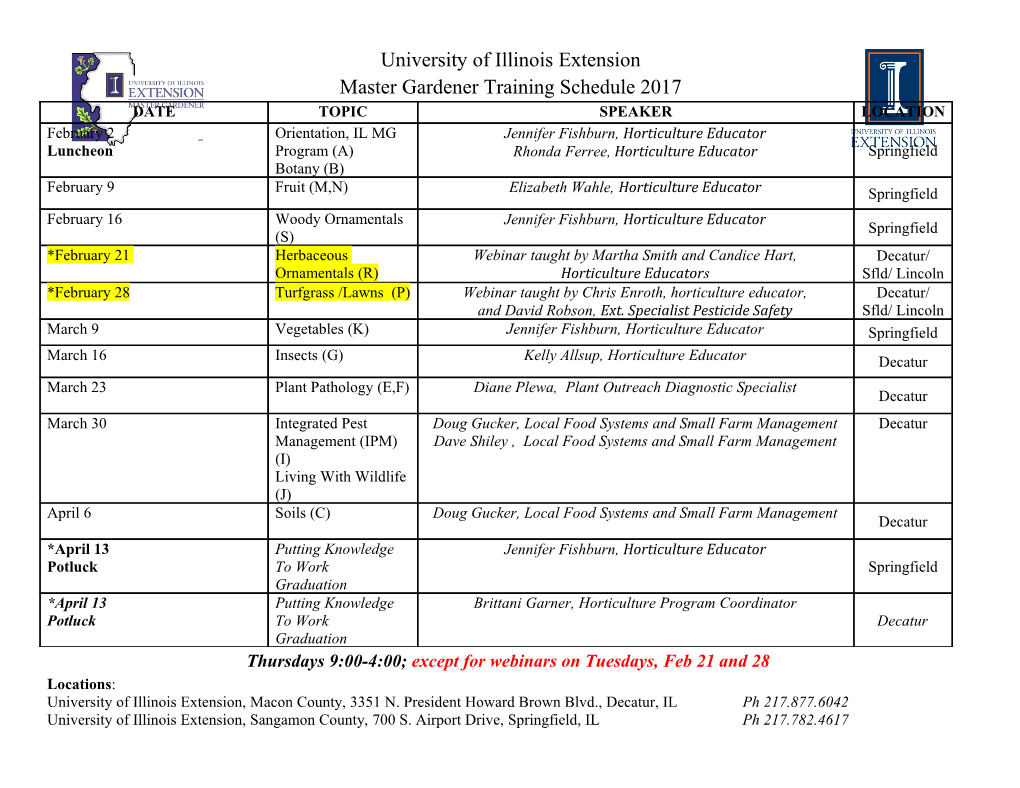
MARINE ECOLOGY PROGRESS SERIES Vol. 74: 1-11, 1991 Published July 18 Mar. Ecol. Prog. Ser. Numerical models show coral reefs can be self -seeding ' Victorian Institute of Marine Sciences, 14 Parliament Place, Melbourne, Victoria 3002, Australia Australian Institute of Marine Science, PMB No. 3, Townsville MC, Queensland 4810, Australia ABSTRACT: Numerical models are used to simulate 3-dimensional circulation and dispersal of material, such as larvae of marine organisms, on Davies Reef in the central section of Australia's Great Barner Reef. Residence times on and around this reef are determined for well-mixed material and for material which resides at the surface, sea bed and at mid-depth. Results indcate order-of-magnitude differences in the residence times of material at different levels in the water column. They confirm prevlous 2- dimensional modelling which indicated that residence times are often comparable to the duration of the planktonic larval life of many coral reef species. Results reveal a potential for the ma~ntenanceof local populations of vaiious coral reef organisms by self-seeding, and allow reinterpretation of the connected- ness of the coral reef ecosystem. INTRODUCTION persal of particles on reefs. They can be summarised as follows. Hydrodynamic experiments of limited duration Circulation around the reefs is typified by a complex (Ludington 1981, Wolanski & Pickard 1983, Andrews et pattern of phase eddies (Black & Gay 1987a, 1990a). al. 1984) have suggested that flushing times on indi- These eddies and other components of the currents vidual reefs of Australia's Great Barrier Reef (GBR) are interact with the reef bathymetry to create a high level relatively short in comparison with the larval life of of horizontal mixing on and around the reefs (Black many different coral reef species (Yamaguchi 1973, 1988). Consequently, the volume of materlal around a 1977, Harrison et al. 1984, Munro & Williams 1985), reef decays exponentially, with the time constant being although Ludington's dye studies in the enclosed a function of net volume flux passing the reef and the lagoon of One Tree Reef suggested residence times of total volume of water on and around the reef (Black et the order of 5 d. Consequently, self-seeding of reefs al. 1990). The former depends on reef size and cross- (i.e.larvae recruiting to the natal reef) has been consid- sectional area perpendicular to the net current. In ered improbable, notwithstanding occasional specula- quantitative terms, for typical weather and current con- tion about possible larval 'black holes' (Cheney 1974, ditions, average-sized reefs can retain larvae for Johannes 1978) and gyres and eddies in the wake of periods in excess of 10 d (Black et al. 1990) even in the reefs and islands (Hammer & Hauri 1981, Wolanski et central GBR. For example, when modelling flow con- al. 1984a, Wolanski et al. 198413). ditions measured during winter 1987, up to 33 % of However, 2-dimensional numerical simulations of neutrally-buoyant material released in the model on the hydrodynamics and dispersal around reefs (Black & John Brewer Reef was still present 10 d later (Gay Gay 1987a, b, 1990a, Black 1988, 1989) have predicted et al. 1990). residence times that are longer than those suggested Furthermore, investigations of the relationship of the by field experiments, after the numerical simulations modelled hydrodynamics to larval dispersal revealed were validated by comparison with a series of detailed that when particles are retained for these durations, field experiments (Wolanski et al. 1984a, Willis & some locations retain more larvae or are visited more Oliver 1988, Wolanski et al. 1989, Gay et al. 1990). often by larvae than others (Black 1988). This was Subsequent studies using the numerical models have confirmed by the results of Willis & Oliver (1988) who made it possible to develop an understanding of the measured a variation in the numbers of coral larvae processes and their interactions which determine dis- around Bowden Reef. Measured larval concentrations O Inter-Research/Printed in Germany 2 Mar Ecol. Prog. Ser 74: 1-11, 1991 at 4 sites around the reef and in the lagoon corre- sponded with those predicted by the model. The high- est concentration of larvae was predicted to occur in the southern compartment of the lagoon. High-fre- quency acoustic soundings subsequently identified a concentration of larvae on the lagoon side of the reef flat (Wolanslu et al. 1989) at the location predicted by At the surface, z = E, the model. Similar correspondence between initial sightings of aggregations of the crown-of-thorns star- fish Acanthasterplanci on individual reefs and concen- trations of larvae predicted by the model was noted by where t = time; U, V = velocities in the X, y directions Black & Moran (1991). respectively at elevation z; W = vertical velocity in the The above findings derive from 2-dimensional mod- z direction (positive upward) at the top of each layer; elling. However, if particles reside only at a particular h = depth; g = gravitational acceleration; 6 = the sea level in the water column, through differences in level above a horizontal datum; f = Coriolis parameter; specific gravity (e.g. Oliver & Willis 1987) or active AH = horizontal eddy viscosity coefficient; and NZ = migration (e.g. Leis 1986), 2-dimensional circulation vertical eddy viscosity coefficient. may not accurately represent passive dispersal. Surface boundary conditions at z = E are Accordingly, a 3-dimensional hydrodynamic and advection/dispersion model was established to assess the importance of 3-dimensional circulation for the residence times of particles representing (1) neutrally where 7,: sySdenote the components of wind stress and buoyant material or (2) material reslding at the surface, mid-depths or near the sea bed. ~x5=Pa~ISISx ~,S=PaYISIS, (6) Thus, the 3-dimensional modelling of a vertically- where p = water density; S = wind speed at 10 m homogeneous water column can be used to test above sea level while S, and S, are the X and y compo- whether different dispersal probabilities exist for nents; y = wind drag coefficient; p, = density of air. organisms with neutrally-buoyant larvae or larvae At the sea bed, z = h, we have which reside at a preferred level, either through specific gravity or behavioural differences. We inter- pret the results of this modelling in relation to the life history characteristics, biology and ecology of some of where zxh, tyl'denote the components of bottom stress. the major groups of coral reef organisms of the GBR. Applying a quadratic law at the sea bed, MODELLING The hydrodynamic model (3DD), a 3-dimensional with Uh, Vh = the bottom currents; and C = Chezy's C. model for vertically homogeneous conditions, is an For a logarithmic profile, extension of the 2-dimensional simulation 2DD (e.g. Black 1983, Black et al. 1989). The 3-dimensional non- linear hydrodynamic equations are solved throughout where z, = roughness length. the depth. In essence, the 3-dimensional model can be The form of the horizontal eddy viscosity term results coded as a series of 2-dimensional models placed on when the depth is presumed constant before taking the top of each other, with the non-linear convective derivative of the horizontal shear stresses. The term, as momentum term and the vertical eddy viscosity linking presented, behaves as a velocity smoothing algorithm the layers. The equations solved are, (Black 1989). A staggered finite difference grid is utilised similar to that applied by Leendertse & Liu (1975) which places the V and U components on 'north and south' walls of each grid box respectively. Wis located in the centre of the 'top' wall. The sea level replaces Win the top layer. The solution is found by time stepping with an explicit solution, as employed in 2DD. Data were not available for a fully stratified, denslty- Black et al.. Numerical models for coral reefs 3 driven coupled simulation (e.g. Black 1990). Thus, the nitude and direction. This was always checked after model is relevant to vertically unstratified hydro- each simulation, confirming that the method was dynamics only. This does not preclude treatment of entirely satisfactory. In addition, a crescentic/lagoonal marine organisms which have a tendency to reside at bathymetry was simulated (l) with a sea gradient favoured levels in the water column. The fully stratified applied at the open boundaries to drive a current and coupled model will await shelf-scale data specifying (2) with the sea gradient replaced with an equivalent the dynamics of such conditions. The measurements of body force. The results obtained showed that the 2 Gay et al. (1990) around John Brewer Reef indicated methods were equivalent, as expected. that significant stratification on the continental shelf The body forces represent the average condition over surrounding the reef was uncommon, although the entire model grid and are calculated using h,,. Wolanski et al. (1989) noted stratification in the lagoon However, as the wind stress is depth-dependent, it is of Bowden Reef and Andrews & Gentien (1982) iden- still necessary to calculate wind stress in each model tified a colder layer of near-bed water on the shelf of cell. The wind stress applied in the cell is the difference the central GBR. Wolanski & Hamner (1988) presented between the average stress incorporated in the body temperature contours in mid-summer from a reef force and the actual stress at the cell in question. Thus, lagoon which showed a temperature difference of 0.6"C between the surface and the bed. Because the 3-dimensional model is composed of a Bed friction, horizontal eddy viscosity and surface wind vertical stack of 2-dimensional simulations, many of the stress were unchanged from the 2-dimensional model techniques applied in the 2-dimensional model (Black runs of Black et al. (1990) and Black (1989).However, a & Gay 1987b) could be used without modification.
Details
-
File Typepdf
-
Upload Time-
-
Content LanguagesEnglish
-
Upload UserAnonymous/Not logged-in
-
File Pages11 Page
-
File Size-