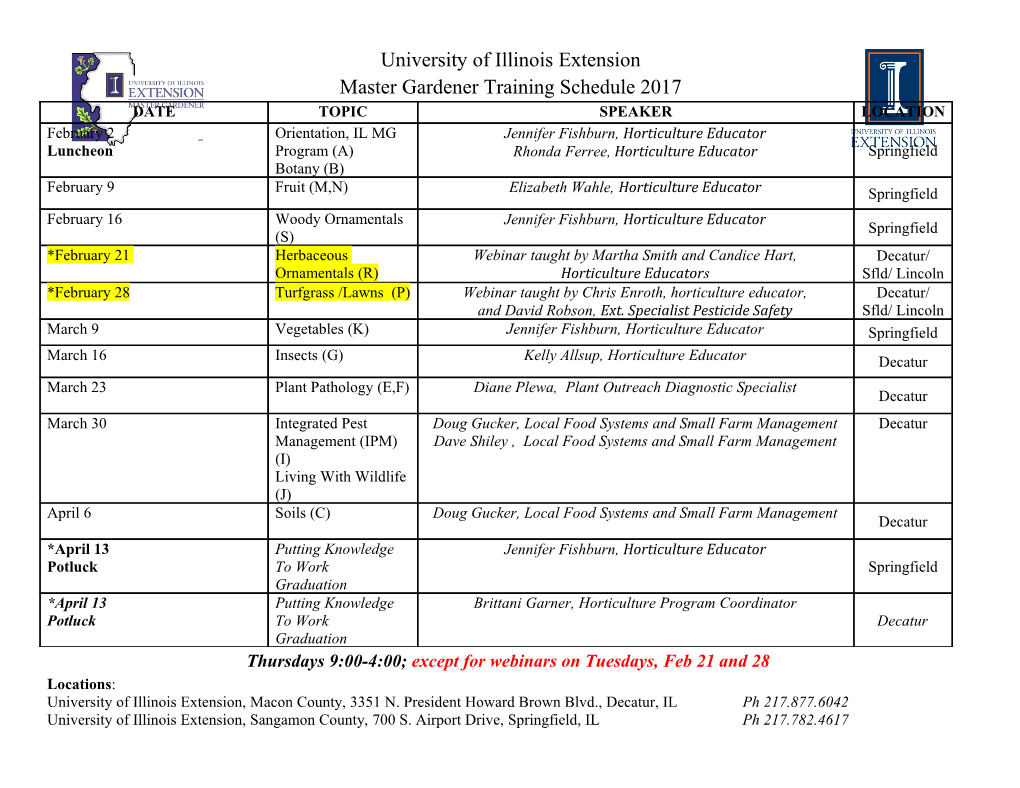
Project ELSA: Europa Lander for Science Acquisition Darren Combs, Gabe Frank, Sara Grandone, Colton Hall, Daniel Johnson Trevor Luke, Scott Mende, Daniel Nowicki, Ben Stringer University of Colorado, Boulder, CO, United States of America Jupiter’s moon Europa is of great interest to the scientific community as evidence suggests there may be a deep liquid water ocean beneath its icy surface. To investigate the surface and subsur- face, future missions to Europa will require the use of landers and/or surface probes to measure surface characteristics and investigate the potentially unique subsurface conditions beneath the ice. Ball Aerospace has begun to explore the feasibility of conducting an exploration with several small, relatively inexpensive probes as an alternative to a large and costly lander. Our team of nine un- dergraduate students has designed and constructed a compact, low-cost, spherical probe, which can collect, store, and transmit scientific data relevant to the study of Europa. The goal of our project was to develop and construct a TRL 4 prototype over the course of 8 months, while keeping the cost under $5000. The development focused on data collection, FPGA software development, and system integration while neglecting environmental elements, such as temperature, radiation, and shocks. Two relevant scientific instruments, a triaxial magnetometer and a Geiger counter, were selected and integrated with an avionics package, which is centered on FPGA-based command and data handling (C&DH). The FPGA is integrated with a transceiver to communicate over RF to a ground station, simulating a Europa orbiter. In order to provide the capability to observe magnetic field and radia- tion variations during one complete orbit of Europa about Jupiter (85 Earth hours), an independent power system was developed and integrated to sustain the probe through its entire mission of 100 hours. The power system, avionics package, science instruments, and transceiver are mounted to a custom-designed structure, contained within a 25.4 centimeter diameter sphere. The prototype collects data for a total of 100 hours, and transmits that data in 8 minute simulated passes, demon- strating the feasibility of using small, cost effective probes to explore the surface of Europa or other planetary bodies. Nomenclature FPGA = Field Programmable Gate Array bps = bits per second TRL = Technology Readiness Level C&DH = Command and Data Handling UHF = Ultra High Frequency BASiX = Binary Asteroid in-Situ Explorer STK = Systems Tool Kit CPM = Counts Per Minute ASCII = American Standard Code for Information Interchange SRAM = Static Random Access Memory RAM = Random Access Memory SPI = Serial Peripheral Interface CR = Current/(5.2×# batteries) (unitless) I. Introduction Europa, one of Jupiter’s four Galilean moons, is believed to have a sizable ocean up to 100 km below its icy surface[1]. NASA has identified Europa as a high priority target in the search for life within our solar system, because of this potential ocean. Spacecraft that have already been sent through the Jovian system have revealed that Europa has one of the smoothest surfaces in the Solar System and has few impact craters which indicates a young and geologically active surface[2]. Photographs of Europa show many large streaks along its surface called lineae. These lineae are suspected to be the result of tidal flexing on Europa’s surface as it orbits Jupiter[3]. In 2013 the Hubble Space Telescope spotted significant plumes of water spouting from the surface, which further suggests that bodies of water exist under 1 of 11 American Institute of Aeronautics and Astronautics the ice. If an ocean does indeed exist, it would be one of the most hospitable places in our Solar System for simple extraterrestrial life. Project ELSA (Europa Lander for Science Acquisition) will provide a stepping stone for future missions exploring Europa by demonstrating the feasibility of collecting relevant data from the surface of Europa within a relatively low cost, low mass, and low volume spherical landing probe, called the NeoPod. The concept of deploying these small, low cost probes to investigate the surface of an astronomical body is rooted in other missions that have been inves- tigated by Ball Aerospace. Previous projects done in conjunction with students from the University of Colorado investigated similar spherical probe systems to deploy to the surface of an asteroid. The student-led TIRESIAS (2013-2014) project developed a fully integrated pod as a building block for the Binary As- teroid in-Situ Explorer (BASiX) mission[4]. The concept for the BASiX mission was to deploy multiple pods to the surface of an asteroid from an orbiter. Once on the surface, one probe carrying an explosive payload would be detonated while other probes measured the vibrational effect. TIRESIAS demonstrated that vibrational data could be collected and transmitted through their commu- Figure 1. Image from orbit nications system with a standalone pod. Ball Aerospace hopes to extend this around Europa concept to the study of Europa by providing an alternative to a large and expensive lander often conceived for a Europa mission. Because of their similarities, a few basic aspects of the TIRESIAS project were re-purposed and integrated into the NeoPod design. The NeoPod has the same outer structure consisting of the aluminum sphere outer casing. The pre- viously developed patch antennas will also be reused in the NeoPod design. However, a significant portion of the project is new and had to be updated or redesigned to fit the Europa specific requirements or to improve flight readiness. Most significant is the payload package, which had to utilize sensors, that if flight-grade, would be capable of collecting meaningful science from the sur- face of Europa. In the TIRESIAS project, avionics were handled using a simple Arduino micro-controller. For this iteration, Ball Aerospace requested the use of a flight-ready avion- ics board with a Field Programmable Gate Array (FPGA). The communications system architecture was improved by using a transceiver chip instead of a sep- Figure 2. NeoPod Design Assem- arate transmitter and receiver package. The power system had to be overhauled bly (one hemisphere removed) to accommodate the longer mission lifetime requirements of 100 hours. And finally, a new internal mounting structure was developed to support the components. With the completion of this project, science data will be collected, processed, and transmitted from the probe, which is a critical step forward to eventually sending scientific probes to Europa. II. Design Objectives This project was driven by three key functional requirements, i) to maximize collection of scientific data relevant to Europa, ii) to have the capability to remotely transmit the data and iii) to fit within specific mission constraints provided by the customer, such as strict weight and volume requirements. The first functional requirement comes from the need to demonstrate that high value science can be done from this kind of platform. While this probe was constructed in a lab environment, future iterations will benefit from the feasibility it demonstrates by collecting scientific data similar to what would be collected on the surface of Europa. The second functional requirement comes from the need to transmit the data once it has been collected. Since the probes will not be physically collected at the end of mission, it is crucial that the NeoPod be able to communicate wirelessly to a simulated orbiter. The overall mission includes a spacecraft orbiting Europa, which is used to collect data from the NeoPod and later transmit back to Earth. As it takes Europa 85 hours to rotate about Jupiter, the mission was designed to survive a full 100 hours. To determine how much data could be collected and sent from the probe from the surface of Europa during this mission, a software simulation of the Europa orbiter was created in Systems Tool Kit (STK). The mission parameters for the Europa orbiter were provided by JPL (95° inclination, 126 minute period, 0 eccentricity, 12° mask angle on probe). Since the orbiter is in a nearly polar orbit, different longitude locations were simulated for the NeoPod probe to rest on the surface which influenced total contact time with the orbiter over 100 hours. Below is a table illustrating the simulated total amount of data that can be transmitted from each latitude of Europa. 2 of 11 American Institute of Aeronautics and Astronautics Transmitted Mission Data at Different Latitudes Over 100 Hour Period Latitude Total Pass Transmit Rate Maximum Maximum Transmittable Data Including Location Time (s) (kbps) Data (MB) 25% Margin (MB) (deg South) 0 2198 128 40 30 20 2094 128 38 29 45 3010 128 55 41 60 4146 128 76 57 75 10149 128 186 139 90 21942 128 401 301 Table 1. STK Scenario Data Return Since the total amount of data return is maximized when the probe is placed at the poles of Europa, the team chose to use this scenario as a design driver. Placing the NeoPod at the poles also functions as a worst case scenario for the power subsystem as the avionics and communications subsystems will perform more computations and tasks over the mission lifetime. The team took these restrictions into mind when designing the communications timeline for the mission by including a realistic, limited window in which it is possible to transmit data. The final two key derived requirements, which flowed from the use of the orbiter, were that the NeoPod shall have a maximum data rate of 128 kbps and that upon command, the NeoPod will begin sending stored data. The team has designed a small Earth-based ground station to simulate this orbiter, which will be used for all testing. The final functional requirement was that the NeoPod needed to fit within existing mission architecture previously designed by Ball Aerospace.
Details
-
File Typepdf
-
Upload Time-
-
Content LanguagesEnglish
-
Upload UserAnonymous/Not logged-in
-
File Pages11 Page
-
File Size-