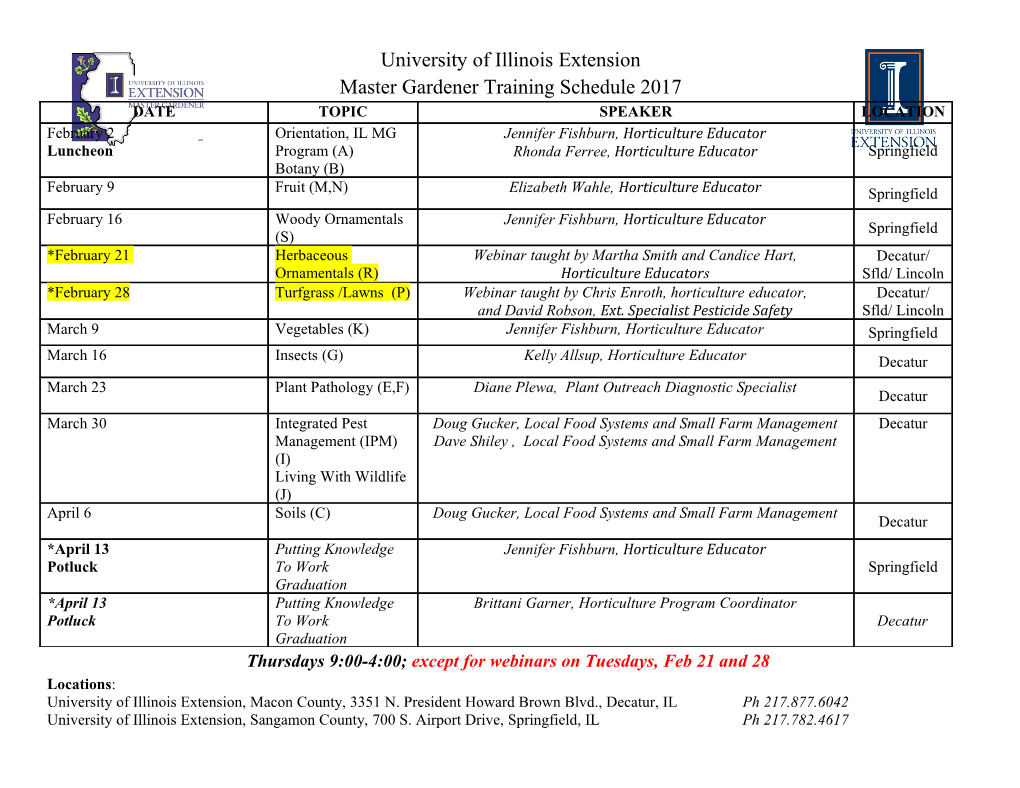
EAS 109, Dinosaurs Text Page 1 of 4 BEFORE THE DINOSAURS: LIFE’S EARLY HISTORY HOW TO MAKE SENSEOF LIFE’ S EVOLUTION — ALL BUT THE DINOSAUR-STUDDEDLAST QUARTER-BILLION YEARS OF IT — IN ONLY 50 MINUTES? First, consider these two helpfully simplifying perspectives on the otherwise impossibly complicated story of life’s evolutionary genealogy: How ecological food chains grew from the bottom up, producers first, top-level carnivores last. How the carbon biogeochemical cycle’s two predominant reservoirs —. Here will be found perhaps the most reassuring evidence discovered to date that all the catastrophes in the Earth’s history, including some that probably dwarfed the K/T event LIFE, THE CARBON CYCLE, AND THE ATMOSPHERE — organic matter enriched in carbon-12 [12C] relative to 13C owing to the lighter isotope’s slightly higher chemical reactivity; of the two main sedimentary carbon reservoirs in the geochemical carbon cycle; as a result, the organic-carbon reservoir is enriched in 12C, and the carbonate-carbon reservoir is correspondingly depleted; the two reservoirs’ carbon-isotopic compositions remain nearly constant throughout life’s 3.5-3.8 Ga of geologically recorded history, indicating that the reservoirs’ relative sizes have remained nearly constant, and that some sort of “balance of nature” has been maintained; buildup of atmospheric oxygen [O2] from photosynthesis, a long and influential trend in the biosphere’s evolution, with O2 becoming a major atmospheric gas in the second half of the Earth’s history; the Gaia hypothesis THE ONGOING REVOLUTION IN OUR UNDERSTANDING OF LIFE (NOT TO MENTION DINOSAURS) Genomics and the related fields of proteomics and bioinformatics seem likely to keep understanding of organisms and their nature, diversity, evolutionary history, and classification in a continuing state of revolution for the foreseeable future. Publication of the more or less complete human genome in 2001 has been hailed as the third millennium’s first major scientific milestone1. Accelerating advances in sequencing DNA, in identifying genes and their protein products, and in comparatively studying organisms’ genomes and proteomes within and between species, promise to revolutionize understanding of organisms’ operation, development, reproduction, and evolution. It has long been realized that organisms’ DNA is a record of their evolutionary history. Since the 1980s it has been possible to read and evaluate microns-long snippets such as the DNA in our own cells’ mitochondria. Now, with computers to process the imposing volumes of data, it has become possible to explore the information encoded in the meter-long DNA molecules in our cell nuclei. So let the reader beware: much that one reads even in current textbooks may be obsolete if not actually wrong; for instance, that we humans have an estimated 100,000 protein-coding genes, as opposed to the 30,000 or so that have now been counted1. CELLS AND ORGANISMS Prokaryotic and Eukaryotic Cells — The characteristics and distinguishing features of these two basic types of cell are tabulated in the accompanying diagram. As can be found on the accompanying geological time scale prokaryotes date back to the earliest indications of like 3.8 Ga ago; fossil eukaryotes, however, date back only about 1.5 Ga, though seeming chemical “fingerprints” of eukaryotes have been identified as much as a billion years earlier. Recent discoveries on archaebacterial prokaryotes and their distinctive biochemical machinery have led many microbiologists to recognize them as a third major group, the Archaea, in distinction to Bacteria (the other prokaryotes) and Eucarya (eukaryotes). 1 The Human Genome, Nature 409, 813-958 (15 February 2001); The Human Genome, Science 291, 1177-1351 (16 February 2001). 9/23/02 EAS 109, Dinosaurs Text Page 2 of 4 Origin of the Eukaryotic Cell — Similarities between the organelles of eukaryotic cells and free-living prokaryotic cells (e.g. organelles’ bacterial-type DNA and ribosomes) suggest that eukaryotes arose as a symbiotic consortium of prokaryotes within an originally prokaryotic host cell, mitochondria developing from aerobic bacteria, chloroplasts from cyanobacteria, and flagella from spirochaetes (as explained in the accompanying diagram). The greater size and anatomical complexity of eukaryotes as compared to prokaryotes evidently corresponds to greater functional specialization and division of labor among the parts of the living cell — a recurring theme in the evolution of organisms at all levels. MULTICELLULAR ORGANISMS Origins of Multicellularity — Multicellularity evidently has arisen at least twice in animals — once in parazoans (sponges) from ancestral flagellate protists, and once in metazoans from probable ciliate ancestors, as indicated in the accompanying phylogenetic tree — and at least once among plants. The eukaryotic cell evidently opened the possibility for cells to differentiate into specialized groups (i.e. tissues) within the organism, opening the possibility for further specialization by division of labor among the organism’s parts (i.e. organs formed of one or more types of tissue). Origin of the Metazoa — Metazoan shells appear rather abruptly in the sedimentary record near the close of the Proterozoic. According to the interpretation depicted in the phylogenetic tree, all the major groups of metazoans appear within roughly 100 Ma. If coral- and jellyfish- like fossils in the Ediacara fauna are indeed coelenterates2, if annelid- or arthropod-like fossils (e.g. Spriggina) are indeed metamerically segmented schizocoelous protostomes, and if vaguely echinoderm-like fossils (e.g. Tribrachidium) are indeed enterocoelous coelomates, then all of today’s major groups of metazoan phyla had differentiated by about 570 Ma ago. Other paleontologists believe that the Ediacara fauna represent a dead-end group that left few if any descendants that may not even have been multicellular. Still others entertain the notion that the variously metazoan-like forms were actually lichens! 2 Here is a brief run-down on important terms and concepts pertaining to metazoan development and phylogeny: Coelenterates (diploblastic metazoans) are characterized as having two cell layers: (i) ectoderm, which originates from the cells of the embryo’s hollow-ball stage (blastula) and eventually becomes the adult body’s outer covering; and (ii) the endoderm, which originates from cells that proliferate inward into the blastula’s interior (blastocoel, the primary body cavity), forming an invagination (the archenteron) that eventually becomes the gut. Triploblastic metazoans, in contrast, have a third cell layer, (iii) the mesoderm, which becomes the musculature, urogenital system, various glands, and other internal organs. In protostomes, the opening into the archenteron’s interior (the blastopore) becomes the mouth, and the mesoderm originates from cells near the blastopore; this is called the schizocoelous pattern of development. In deuterostomes, on the other hand, the blastopore becomes the anus, and the mesoderm originates near the other end of the archenteron; this is called the enterocoelous pattern of development. Whichever end of the digestive tract has formed first, the other end breaks through later in development (if it is going to do so at all). Triploblastic metazoans may have no secondary body cavities, in which case they are called acoelomates; they may have a secondary body cavity developed between the inner and outer layers of mesoderm known (the coelom in the strict sense of the term), in which case they are called coelomates; or they may have a secondary body cavity developed in some other way, in which cases they are called pseudocoelomates. A true coelom evidently evolved at least twice, once among protostomes (the schizocoelomates), and once among deuterostomes (the enterocoelomates), and each time, it appears, in conjunction with the evolution of metameric segmentation (i.e. serial repetition of body parts based on early embryonic segmentation of the coelom). 9/23/02 EAS 109, Dinosaurs Text Page 3 of 4 The evidence that the sudden appearance of metazoans’ shells in the fossil record signifies the appearance of metazoans themselves, and not simply the appearance of shells in metazoan lineages of much greater antiquity, is that trace fossils and bioturbation first appear in the sedimentary record no more than 1-10 Ma before the earliest known shells. In many if not most lineages, development of a durable shell seems to have been related to the demand for skeletal support that accompanies increase in size3. Somewhat contrary to evidence of fossils and sediments, evidence of molecular evolution suggests a divergence among metazoan phyla as far back of 1000 Ma when interpreted in light of evolutionary rates in certain genes and 18S ribosomal RNA4. The implication is that metazoans appeared and diversified as more or less microscopic forms and then diversified again as adaptations for larger size evolved more or less independently in already-differentiated lineages as much as half a billion years later. This conclusion, however, is based on the assumption that evolution’s “molecular clock” has ticked at a constant rate — an assumption that quite conceivably may not have applied while the clockworks themselves were undergoing significant evolutionary change, as they likely were during the evolution of metazoans’ radical evolutionary innovation: sex, and with it, vast new possibilities for generating genetic diversity. To recap, not all protostomes are coelomates,
Details
-
File Typepdf
-
Upload Time-
-
Content LanguagesEnglish
-
Upload UserAnonymous/Not logged-in
-
File Pages4 Page
-
File Size-