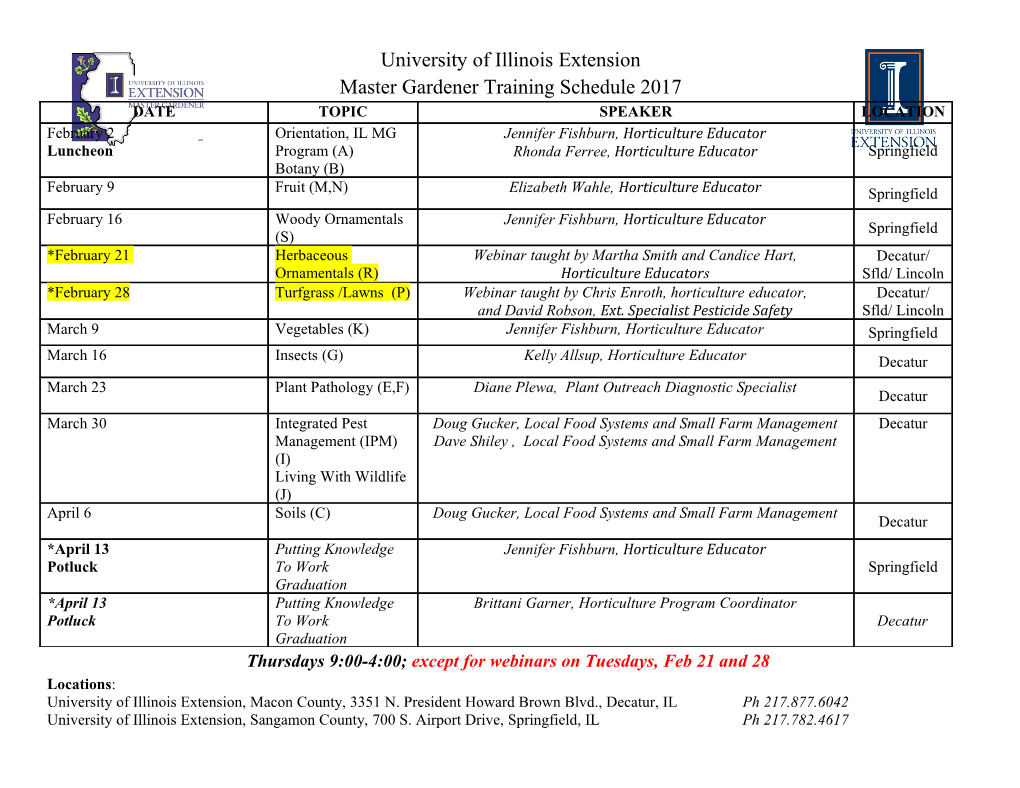
1 1 Structure of the Bacterial Cell All bacterial cells are surrounded by at least one membrane, the cytoplasmic membrane enclosing the cytoplasm. This simple enclosure can be found only by species living within eukaryotic cells such as Mycoplasma spp. But most cells are surrounded in addition by a thick cell wall (the Gram-positives) and another group by a thin cell wall followed by a second membrane, the outer membrane (the Gram-negatives), where both membranes are separated by the periplasm. Further- more, cells can be surrounded by an extracellular matrix, a capsule or an S-layer, a monomolecular protein layer. In total, Gram-positive bacteria consist of three compartments, the cytoplasm, the cytoplasmic membrane and the extracyto- plasm, while Gram-negatives contain two additional compartments, the peri- plasm and the outer membrane. In addition, cells can contain appendages, flagel- la and/or pili allowing movements and attachment to cell surfaces, respectively. We will present an overview of the composition and function of these different compartments. For details, classic microbiology textbooks should be consulted. 1.1 The Cytoplasm Compartment First of all, the cytoplasm contains all the different types of nucleic acids: the chro- mosome(s) and quite often one or more plasmids and the arsenal of RNAs (mRNA, rRNA, tRNA, sRNA). In addition, the cytoplasm consists of an aqueous solution of salts, sugars, amino acids, vitamins, coenzymes and a wide variety of other soluble materials including about 2000 different protein species. The cyto- plasmic proteins fold into structures that place their hydrophobic segments in the interior to reduce their entropic cost of their exposure to water, and polar residues are generally placed on the protein surface where they can be hydrated. Tradi- tional textbook representations of the prokaryotic cytoplasm show an amorphous amalgamation of proteins in which a randomly arranged chromosome is located. This image of the microbial cell is not correct and, instead, the cytoplasm is highly structured with many proteins carrying out their assigned functions at specific subcellular locations. Therefore, the bacterial cell is more than a bag of proteins. While most proteins may be dispersed throughout the cytoplasm, some seem to Dynamics of the Bacterial Chromosome. Structure and Function. W. Schumann Copyright g 2006 Wiley-VCH Verlag GmbH & Co. KGaA ISBN 3-527-30496-7 2 1 Structure of the Bacterial Cell have specific localizations. One example is the B. subtilis replisome which is pre- sent throughout replication of the chromosome within the middle of the cell. Pro- teins can be localized within the cell through a so-called localization tag which is fused either to the N- or to the C-terminus. The most prominent tag is GFP (for green fluorescent protein) and its variants. Furthermore, all macromolecular pro- cesses occur in the cytoplasm, such as DNA replication, transcription, translation and recombination. The Bacterial Cytoskeleton All eukaryotic cells, from yeast to plants to animals, have an internal framework called the cytoskeleton. The three types of strut that make up this framework ± microtubules, actin filaments and intermediate filaments ± not only provide cells with mechanical support, but also serve as tracks for motor molecules to move along. Bacteria were long thought to lack cytoskeletal filaments, suggesting that the cytoskeleton might have evolved after the first primitive eukaryotic cell devel- oped from its bacterial origins. But work carried out over the past few years has shown that bacteria contain cytoskeletons with homologs to all the eukaryotic cy- toskeletal elements. Bacteria contain a microtubule (tubulin) protein called FtsZ, multiple actin-like proteins including MreB and Mbl, and intermediate filament proteins such as crescentin in C. crescentus, indicating the very ancient origin of these types of intracellular filaments. Strikingly, no nucleators or motor proteins have been identified for any bacterial cytoskeletal element. In eukaryotes, microtu- bules are involved in various transport processes and, in forming the mitotic spin- dle during chromosome segregation, actin forms a dynamic cytoskeleton involved in cell shape regulation and mobility as well as the cytokinetic ring during cell di- vision; and intermediate filaments provide mechanical support to the cell and nu- cleus. In procaryotes, the tubulin homolog FtsZ is essential for cell division, form- ing a cytokinetic ring at mid-cell early during the division process (see Section 3.3). In association with a number of other cell division proteins, FtsZ then con- stricts concomitantly with septal peptidoglycan synthesis to bisect the cell. The two actin-like proteins, MreB and Mbl, in B. subtilis form helical filaments. The two proteins do not interact to form a hybrid filament but each protein forms a fi- lament of different pitch and length. While MreB forms a short (0.730.12 ìm) pitch filament that assembles around the mid-cell position, Mbl filaments (half- life ;8 min) have a longer pitch (1.70.28 ìm) and traverse the entire cell length. MreB spirals are dynamic throughout the cell cycle, compacting at the division plane in predivisional cells, remaining there until division is complete and then expanding to fill the cell. MreB has a role in the maintenance of the cell width and plays a role in chromosome segregation, whereas Mbl is involved in the mainte- nance of the long axis of the cell. Depletion of either MreB or Mbl causes aberrant partitioning of the origins of replication and a large fraction of anucleate cells. The intermediate filament crescentin (encoded by creS) of C. crescentus is closely juxtaposed with the cell membrane and is responsible for the vibrioid shape: cell lacking crescentin are rod-shaped. CreS filaments represent true architectural 1.2 The Cytoplasmic Membrane Compartment 3 structures within the cell, as their conformation dictates cell shape. And the ParM protein encoded by plasmid R1 forms F-actin-like cables that actively move plas- mid DNA. In 2001, the three-dimensional structure of MreB of T. maritima was solved and revealed that it is congruent with that of eukaryotic actins. Later, the structure of a plasmid-encoded ParM protein was solved and shown to be very si- milar to that of actin and MreB. Both proteins and several others, such as Mbl of B. subtilis, now belong to the MreB family; and these proteins carry out nucleotide binding and hydrolysis which regulates polymerization into linear protofilaments. 1.2 The Cytoplasmic Membrane Compartment The cytoplasmic membrane is an 8 nm thick structure enclosing the cytoplasm, separating the interior of the cell from the environment and preventing the diffu- sion of substances into and out of the cytoplasm. It acts as a highly selective bar- rier to concentrate metabolic compounds and nutrients within the cytoplasm and to secrete waste products. Structure and Composition of the Cytoplasmic Membrane The cytoplasmic membrane consists of equal amounts of phospholipid and pro- tein. It contains 65±75% of the cellular phospholipids and 6±9% of the cellular protein. The phospholipids consist of a hydrophobic part (fatty acids) and a hydro- philic part (glycerol), where the glycerol backbone contains two bound fatty acids and a phosphate group. Three major phospholipid species are present in E. coli, amounting to about 2¥107 molecules per cell: 70±80% phosphatidylethanolamine, 15±25% phosphatidylglycerol and 5±10% cardiolipin. All of the phospholipids contain sn-glycerol-3-phosphate esterified with fatty acids at the sn-1 and sn-2 posi- tions. The predominant fatty acids are the saturated palmitic acid (16:0) and the unsaturated species palmitoleic acid (16:1) and cis-vaccenic acid (18:1). The struc- ture of the membrane is stabilized by hydrogen bridges and hydrophobic interac- tions; and, additionally, cations such as Mg2+ and Ca2+ help to stabilize the mem- brane by forming ionic bonds with the negative charges of the phospholipids. Fatty acid content (chain length, saturation) are dependent on the environmental conditions, including temperature (see Section 9.3), stage of growth and composi- tion of the growth medium. In particular, membranes have to be maintained in a fluid, liquid±crystalline state to allow lateral diffusion of their proteins and protein complexes. In addition, fluid membranes have much higher permeabilities to small molecules than do gel-phase bilayers. The phospholipids arrange in a lipid bilayer, forming a hydrophobic barrier, preventing the uncontrolled movement of polar molecules and allowing the retension of metabolites and proteins. While some proteins are tightly bound to the membrane with one or more membrane-spanning domains (so-called integral membrane proteins), others are loosely bound (peripheral membrane proteins) or interact only transiently. Bioin- 4 1 Structure of the Bacterial Cell formatic analysis of the E. coli proteome indicates that approximately 1000 of the 4288 predicted genes encode integral membrane proteins that are critically impor- tant for many cellular functions. However, owing to their hydrophobic and amphi- philic nature, membrane proteins are difficult to study; and they account for less than 1% of the known high-resolution protein structures. Topology models de- scribe the number of transmembrane spans and the orientation of the protein re- lative to the lipid bilayer. For E. coli proteins, location of their C-terminus can be easily determined through the use of the topology reporter proteins alkaline phos- phatase (PhoA) and GFP. PhoA and GFP have opposite activity proliles: PhoA is active only in the periplasm, whereas GFP is fluorescent only in the cytoplasm. When fused to the C-terminus of a membrane protein, PhoA and GFP accurately report on which side of the membrane the C-terminus is located. The distribution of the membrane proteins changes considerably under different medium and growth conditions. The amino acid residues of membrane proteins in the interior have similar hydrophobic character, as residues in the interior of the soluble pro- teins and the residues that are exposed to the aqueous environment have the ex- pected polar character.
Details
-
File Typepdf
-
Upload Time-
-
Content LanguagesEnglish
-
Upload UserAnonymous/Not logged-in
-
File Pages28 Page
-
File Size-