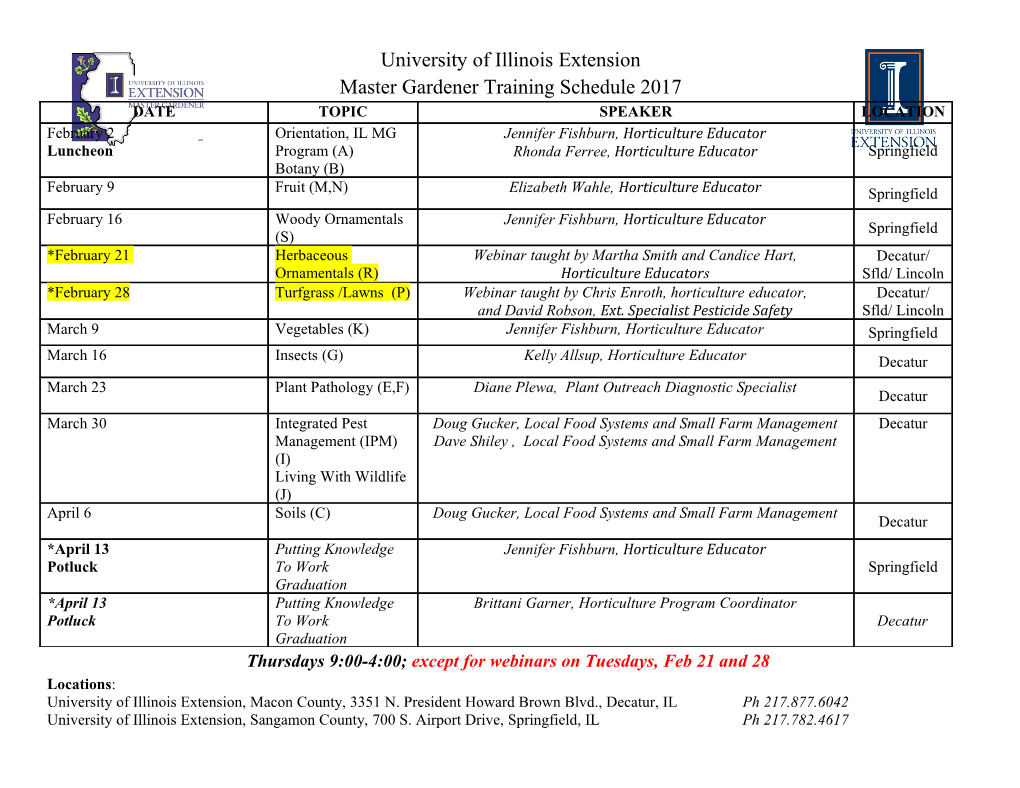
biology Review Epigenetics for Crop Improvement in Times of Global Change Ioanna Kakoulidou 1, Evangelia V. Avramidou 2 , Miroslav Baránek 3 , Sophie Brunel-Muguet 4, Sara Farrona 5, Frank Johannes 1,6, Eirini Kaiserli 7 , Michal Lieberman-Lazarovich 8, Federico Martinelli 9 , Velimir Mladenov 10 , Pilar S. Testillano 11 , Valya Vassileva 12 and Stéphane Maury 13,* 1 Department of Molecular Life Sciences, Technical University of Munich, Liesel-Beckmann-Str. 2, 85354 Freising, Germany; [email protected] (I.K.); [email protected] (F.J.) 2 Laboratory of Forest Genetics and Biotechnology, Institute of Mediterranean Forest Ecosystems, Hellenic Agricultural Organization-Dimitra (ELGO-DIMITRA), 11528 Athens, Greece; [email protected] 3 Faculty of Horticulture, Mendeleum—Institute of Genetics, Mendel University in Brno, Valtická 334, 69144 Lednice, Czech Republic; [email protected] 4 UMR 950 Ecophysiologie Végétale, Agronomie et Nutritions N, C, S, UNICAEN, INRAE, Normandie Université, CEDEX, F-14032 Caen, France; [email protected] 5 Plant and AgriBiosciences Centre, Ryan Institute, National University of Ireland (NUI) Galway, H91 TK33 Galway, Ireland; [email protected] 6 Institute for Advanced Study, Technical University of Munich, Lichtenberg Str. 2a, 85748 Garching, Germany 7 Institute of Molecular, Cell and Systems Biology, College of Medical, Veterinary and Life Sciences, Bower Building, University of Glasgow, Glasgow G12 8QQ, UK; [email protected] 8 Institute of Plant Sciences, Agricultural Research Organization, Volcani Center, Rishon LeZion 7505101, Israel; [email protected] 9 Department of Biology, University of Florence, 50019 Sesto Fiorentino, Italy; federico.martinelli@unifi.it 10 Faculty of Agriculture, University of Novi Sad, Sq. Dositeja Obradovi´ca8, 21000 Novi Sad, Serbia; Citation: Kakoulidou, I.; Avramidou, [email protected] 11 E.V.; Baránek, M.; Brunel-Muguet, S.; Pollen Biotechnology of Crop Plants Group, Centro de Investigaciones Biológicas Margarita Salas-(CIB-CSIC), Farrona, S.; Johannes, F.; Kaiserli, E.; Ramiro Maeztu 9, 28040 Madrid, Spain; [email protected] 12 Lieberman-Lazarovich, M.; Martinelli, Department of Molecular Biology and Genetics, Institute of Plant Physiology and Genetics, Bulgarian Academy of Sciences, Acad. Georgi Bonchev Str., Bldg. 21, 1113 Sofia, Bulgaria; F.; Mladenov, V.; et al. Epigenetics for [email protected] Crop Improvement in Times of 13 Laboratoire de Biologie des Ligneux et des Grandes Cultures, INRAE, EA1207 USC1328, Biology 10 Global Change. 2021, , 766. Université d’Orléans, F-45067 Orléans, France https://doi.org/10.3390/ * Correspondence: [email protected]; Tel.: +33-2-38-41-70-22 biology10080766 Simple Summary: Research on plant epigenetics aims to understand how endogenous, biotic, and Academic Editors: Pierre Devaux and abiotic factors regulate plant development and growth independent of changes in the genome Pierre Sourdille sequence. Often, the epigenetic changes are heritable across generations and modulate plant growth and crop tolerance, particularly in response to environmental stimuli. To take advantage of epigenetic Received: 29 June 2021 Accepted: 6 August 2021 adaptation, recent research has focused on implementing targeted epigenetic diversity to engineer Published: 11 August 2021 plants that harbour advantageous traits for optimal crop production. Epigenetics has the potential to provide a powerful toolbox for crop breeders; however, most mechanistic studies are based on Publisher’s Note: MDPI stays neutral information from model plant species due to the challenges that arise when working with crops. with regard to jurisdictional claims in Here, we summarise the contribution of epigenetics to optimising crop adaptation in response to published maps and institutional affil- climate change and overview potential future applications as well as challenges. iations. Abstract: Epigenetics has emerged as an important research field for crop improvement under the on-going climatic changes. Heritable epigenetic changes can arise independently of DNA sequence alterations and have been associated with altered gene expression and transmitted phenotypic varia- Copyright: © 2021 by the authors. tion. By modulating plant development and physiological responses to environmental conditions, Licensee MDPI, Basel, Switzerland. epigenetic diversity—naturally, genetically, chemically, or environmentally induced—can help opti- This article is an open access article mise crop traits in an era challenged by global climate change. Beyond DNA sequence variation, the distributed under the terms and epigenetic modifications may contribute to breeding by providing useful markers and allowing the conditions of the Creative Commons use of epigenome diversity to predict plant performance and increase final crop production. Given Attribution (CC BY) license (https:// the difficulties in transferring the knowledge of the epigenetic mechanisms from model plants to creativecommons.org/licenses/by/ crops, various strategies have emerged. Among those strategies are modelling frameworks dedicated 4.0/). Biology 2021, 10, 766. https://doi.org/10.3390/biology10080766 https://www.mdpi.com/journal/biology Biology 2021, 10, 766 2 of 46 to predicting epigenetically controlled-adaptive traits, the use of epigenetics for in vitro regeneration to accelerate crop breeding, and changes of specific epigenetic marks that modulate gene expression of traits of interest. The key challenge that agriculture faces in the 21st century is to increase crop production by speeding up the breeding of resilient crop species. Therefore, epigenetics provides fundamental molecular information with potential direct applications in crop enhancement, tolerance, and adaptation within the context of climate change. Keywords: breeding; climate change; DNA methylation; epigenomics; memory; plant epigenetics; prediction models; priming 1. Introduction Based on the Paris Agreement in 2015, the United Nations agreed to limit global warming to 2.0 ◦C with the ambition to attempt to cap warming at 1.5 ◦C (UNFCCC, 2015). Greenhouse gases (GHGs) released from human activities are universally recognised as the most significant driver of shifts in climate change observed since the mid-20th century (IPCC, 2013). Agriculture, forestry, and other land uses are responsible for almost a quarter of anthropogenic GHG emissions [1], and a reduction in agriculture-derived emissions is essential for limiting global warming [2]. On the other hand, numerous studies have shown that agricultural crop production and food security is one of many sectors already affected by climate change [3–5]. Recent reports on the impact of climatic trends on wheat, maize, and barley yields based on new statistical and empirical models revealed a negative response of global yields to increased temperatures [6,7]. Besides global warming, biotic constraints, such as pathogens, pests, and weeds, can be detrimental to crop production worldwide. Numerous studies on different crops, such as soybean [8], have revealed the effect of pathogens, pests, and insects on annual soybean production worldwide, and on wheat and cotton, where pest attacks are responsible for damaging more than 50% or 80% of the annual production [9]. A global strategy to minimise major crop losses by optimising crop protection and resilience in response to biotic and abiotic stresses is essential for safeguarding future food availability [10]. To face these biotic and abiotic constraints, emergence for improved adaptation and selection of superior genotypes through the study of epigenetics will have a significant impact on future crop sustainability. In eukaryotes, including plants, genomic DNA is tightly packaged into a dynamic but stable nucleoprotein complex, known as chromatin, whose conformation enables DNA accessibility and controls all DNA-based activities [11]. The primary structural unit of chromatin compaction is the nucleosome, comprising DNA wrapped around a core of eight highly conserved histone molecules. The level of nucleosome occupancy orchestrates chromatin accessibility to the transcriptional machinery and to other regulatory proteins, thus affecting gene availability for transcription. In this context, “epigenetic” can be de- fined as mitotically and/or meiotically heritable changes in gene function that cannot be explained by changes in DNA sequence. Then, modulators of chromatin compaction are regarded as epigenetic marks, including DNA methylation, histone modifications, chromatin remodelers, and to some extent small RNAs [12]. DNA methylation is defined by the covalent addition of a methyl group (CH3) to the fifth position of a cytosine ring (5 mC) by DNA methyltransferases without altering the DNA sequence [13–15]. His- tone variants and post-translational modifications (PTMs) [16], such as phosphorylation, acetylation, and methylation, are essential elements of the chromatin signalling path- way. Lastly, RNA molecules are either small RNAs (small interfering RNAs-siRNAs and microRNAs-miRNAs) or long non-coding RNAs (long ncRNAs) and have been demon- strated to contribute to phenotypic changes. All these epigenetic marks are closely linked, acting together to coordinate gene activity at the transcriptional level, and regulate dif- ferent cellular processes, such as DNA replication and repair, stem cell maintenance, the establishment of cell identity, and tissue and organ development and differentiation,
Details
-
File Typepdf
-
Upload Time-
-
Content LanguagesEnglish
-
Upload UserAnonymous/Not logged-in
-
File Pages46 Page
-
File Size-