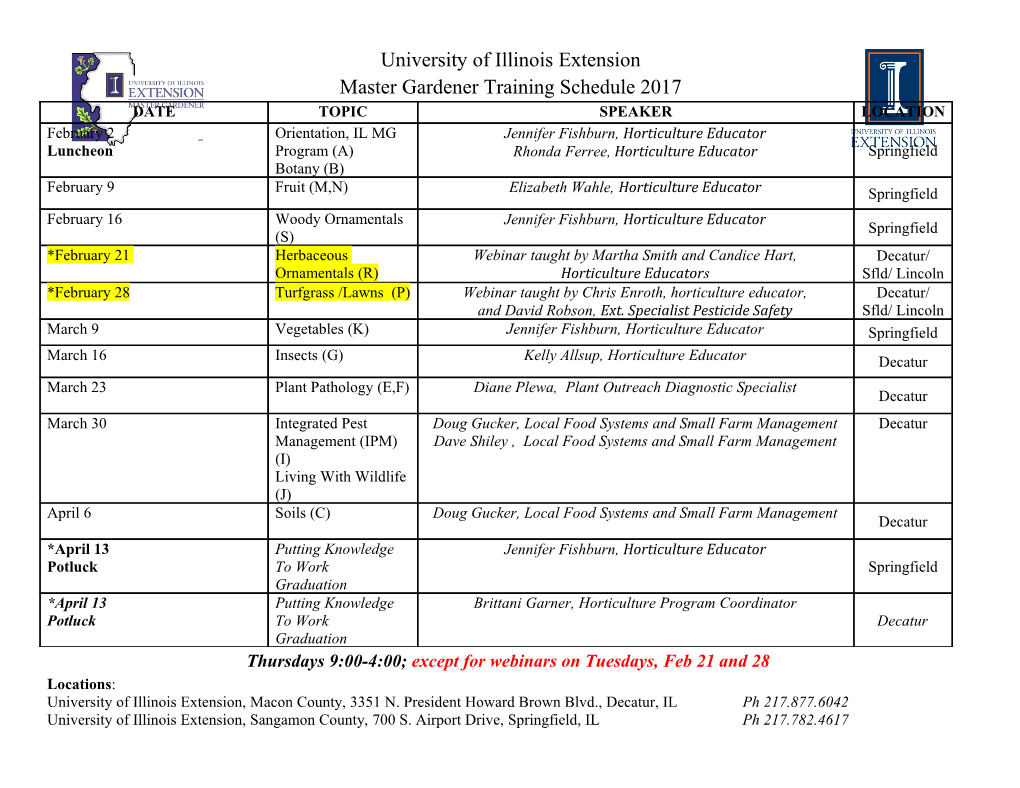
J. Am. Chem. Soc. 1993, 115, 751-755 751 A New Catalysis for Benzene Production from Acetylene under UHV Conditions: Sn/Pt( 111) Surface Alloys Chen Xu, John W. Peck, and Bruce E. Koel* Contribution from the Department of Chemistry, University of Southern California, Los Angeles, California 90089-0482. Received July 23, 1992 Abstract: The adsorption and reaction of acetylene on Pt(l 11) and the p(2X2) and (v/3Xv/3)R30°Sn/Pt(l 11) surface alloys were investigated with low energy electron diffraction (LEED), temperature programmed desorption (TPD), and Auger electron spectroscopy (AES). The presence of Sn atoms at the surface of the p(2X2) and (v/3Xv/3)R30°Sn/Pt(l 11) surface alloys strongly suppressed the decomposition of acetylene (C2D2) to deuterium and adsorbed carbon. As a result, a new reaction path is opened on the Sn/Pt(l 11) surface alloys—benzene formation. Besides benzene desorption, we also observed butadiene desorption, which is obviously a C4 product of a stable intermediate in benzene production. The (\/3X\/3)R30oSn/Pt(l 11) surface shows the highest activity and selectivity for the formation of benzene and butadiene. Following C2D2 adsorption on the Pt(l 11) surface at 110 K, LEED shows a faint (2X2) pattern. After saturation dosing of acetylene on the p(2X2)Sn/Pt(l 11) surface at 110 K we find a large increase in the (2X2) LEED pattern intensity. This implies that an acetylene (2X2) substructure also forms on the p(2X2)Sn/Pt(l 11) surface. Introduction adsorption on Sn/Pt(l 11) surface alloys have shown that the alloys The palladium-catalyzed cyclotrimerization of acetylene has have a greatly reduced reactivity for hydrocarbon dehydrogenation attracted a lot of attention because of the measurable amount of on clean Pt(l 11).1314 Thus, we were attracted to explore acetylene benzene formation found even under ultra-high-vacuum (UHV) adsorption and reaction on Sn/Pt(l 11) surface alloys. We find conditions. Lambert and co-workers1'8 have led the way in in- that the decomposition of acetylene is strongly suppressed with vestigations of this system. A general mechanism for this reaction increasing presence of Sn at the surface. As a result, benzene has been established which involves a sequential process (C2 -* formation is promoted. Benzene and butadiene desorption is C4 — C6), without either C-H or C-C bond cleavage. The C4 observed under UHV conditions below 400 K. intermediate was first identified DC beam by experiments.1 Experimental Methods Further comes from resolution electron support indirectly high The experiments were performed in a stainless steel UHV chamber. loss measurements of energy spectroscopy (HREELS) C4H4 The system is equipped with instrumentation for Auger electron spec- species produced by dissociative chemisorption of C4H4C12.4 troscopy (AES) and low energy electron diffraction (LEED), a shielded In contrast to Pd, the transition metals in the same group, Ni UTI quadrupole mass spectrometer (QMS) for temperature programmed and Pt, do not show any reactivity for the cyclotrimerization of desorption (TPD), and a directed beam doser for making sticking coef- acetylene under UHV conditions. The decomposition of acetylene ficient measurements. The system base pressure is 6 X 10'11 Torr. TPD were made the in with the to hydrogen and adsorbed carbon is the only reaction pathway measurements using QMS line-of-sight sample surface and a linear rate of 4-5 The observed. This is generally thought to be due to the higher re- using heating K/s. Pt( 111) crystal can be cooled down to 95 K using liquid nitrogen or resistively heated activity of Pt and Ni compared to Pd. On a Pt(l 11) surface below to 1200 K. The temperature was measured by a chromel-alumel ther- 330 adsorbs in a K, acetylene M3-’?2 configuration by rehybridi- mocouple spotwelded to the side of the crystal. zation of the carbon atoms to 5. Between 330 and 400 K ~sp2 The Pt(l 11) crystal was cleaned using the procedure found in ref. 15. adsorbed acetylene disproportionates to an adsorbed bridging The p(2X2)Sn/Pt(l 11) and p(v'3Xv'3)R30oSn/Pt(l 11) surface alloys ethylidyne (CCH3) and an unidentified residue, possibly C2H, were prepared by evaporating Sn on the clean Pt( 111) surface and sub- which decompose further to hydrogen and carbon with heating sequently annealing them to 1000 K for 10 s. Depending upon the initial to 800 K. Sn coverage, the annealed surface exhibited a (2X2) or (v/3xv/3)R30° LEED for these has been Pt-Sn bimetallic catalysts are becoming increasingly important pattern. The structure patterns assigned to the face of and a substitutional surface of Downloaded via PRINCETON UNIV on March 19, 2021 at 20:10:44 (UTC). for catalytic reforming.9'12 In one type of reaction in the re- (111) Pt3Sn alloy composition Pt2Sn,16 as shown in Figure 1. Angle-dependent low energy ion scat- forming process, saturated hydrocarbons are converted to aromatic tering spectroscopy (LEISS) measurements using 500-1000 eV Li+ as as because of the an- hydrocarbons selectively possible high clearly show that surface alloys (rather than Sn adatoms) are produced tiknock of aromatic Pt-Sn be quality hydrocarbons. alloys may and that Sn atoms are almost coplanar with the Pt atom at the surface; See https://pubs.acs.org/sharingguidelines for options on how to legitimately share published articles. formed under certain conditions on supported Pt-Sn bimetallic Sn only protrudes ~0.022 ± 0.005 nm above the surface.17 This in- catalysts,10 and these alloys may be responsible for the selective dicates a strong interaction between Sn and Pt atoms. Hereafter, we will aromatization reactions. Recent investigations of organic molecule denote these surfaces as the (2X2) or v/3Sn/Pt(l 11) surface alloys. Because of the carbon contamination resulting from each adsorption and TPD experiment and the strong affinity of oxygen for Sn, the Sn/ (1) Tysoe, W. T.; Nyberg, G. L.; Lambert, R. M. Surf. Sci. 1983,135, 128. Pt( 111) surface alloys must be newly prepared for each experiment. Sn (2) Patterson, C. H.; Lambert, R. M. J. Phys. Chem. 1988, 92, 1266. was removed at 1000 K for 15 min. The (3) Patterson, C. H.; Lambert, R. M. J. Am. Chem. Soc. 1988,110, 6871. easily by sputtering sputtered surface was then heated at 800 K in 2 X 10'8 Torr of and annealed (4) Patterson, C. H.; Mundenar, J. M.; Timbrell, P. Y.; Gellman, A. J.; 02 Lambert, R. M. Surf. Sci. 1989, 208, 93. to 1200 K. The cleanliness was checked by AES. (5) Ormerod, R. M.; Lambert, R. M. J. Chem. Soc., Chem. Commun. Acetylene (C2D2) was used as received from Cambridge Isotope 1990, 1421. Laboratories (CIL) and the purity was checked by in situ mass spec- (6) Hoffman, H.; Zaera, F.; Ormerod, R. M.; Lambert, R. M.; Wang, L. trometry. The sticking coefficient of acetylene was determined using a W. T. Sci. 259. P.; Tysoe, Surf. 1990, 232, simple kinetic uptake method which was described previously.18 The (7) Ormerod, R. M.; Baddeley, C. J.; Lambert, R. M. Surf. Sci. 1991, 259, L709. (8) Hoffman, H.; Zaera, F.; Ormerod, R. M.; Lambert, R. M.; Yao, J. M.; (13) Paffett, M. T.; Gebhard, S. C.; Windham, R. G.; Koel, B. E. Surf. Saldin, D. K.; Wang, L. P.; Bennett, D. W.; Tysoe, W. T. Surf. Sci. 1992, Sci. 1989, 223, 449. 268, 1. (14) Xu, C.; Koel, B. E. to be submitted for publication. (9) Sinfelt, J. H. Bimetallic Catalysis'. Discoveries, Concepts, and Ap- (15) Campbell, C. T.; Ertl, G.; Kuipers, H.; Segner, J. Surf. Sci. 1981, 107, plications', Wiley: New York, 1983; pp 130-157. 220. (10) Meitzner, G.; Via, G. H.; Lytle, F. W.; Fung, S. C.; Sinfelt, J. H. J. (16) Paffett, M. T.; Windham, R. G. Surf. Sci. 1989, 208, 34. Phys. Chem. 1988, 92, 2925. (17) Overbury, S. H.; Mullins, D. R.; Paffett, M. T.; Koel, B. E. Surf. Sci. (11) Srinivasan R.; Rice, L. A.; Davis, B. H. J. Catal. 1991, 129, 257. 1991, 254, 45. (12) Balakrishnan, K.; Schwank, J. J. Catal. 1991, 132, 451. (18) Jiang, L. Q.; Koel, B. E.; Falconer, J. L. Surf. Sci. 1992, 273, 273. 0002-7863/93/1515-751 $04.00/0 &copy; 1993 American Chemical Society 752 J. Am. Chem. Soc., Vol. 115, No. 2. 1993 Xu el al. (V3XV3)R30° Pt2Sn 0Sn»O.33 Figure 2. C2D2 TPD spectra after acetylene saturation exposure on the Pt(l 11) surface (bottom curve), (2X2)Sn/Pt(lll) surface alloy (middle curve), and (\/3X\/3)R30<’Sn/Pt(l 11) surface alloy (top curve) at 110 K. Figure 1. Structures for the (2X2) and (v'3Xv'3)R30® Sn/Pt(tll) surface alloys. acetylene coverage, 6, was calibrated with the following equation,1'' using the well-known saturation coverage of CO, 0CO = 0.5," on clean Pt( 111) at 300 K: dt f'ltei, k->t / \ § -'jcetylenc 0) 7*CO \ ^ictiylene / J Sqq dt Adsorbate coverages in this paper are referenced to the Pt( 111) sur- = face atom density, i.e., 9 1 is defined as 1.505 X 10" cm Results and Discussion Acetylene Adsorption. The thermal desorption spectra of molecular acetylene and D, evolution after the Pt( 111) and Sn/Pt(l 11) surface alloys are saturated with acetylene are sum- marized in Figures 2 and 3, The TPD spectra from clean Pt( 111) are in good agreement with data published previously.20-22 Using the TPD area we estimate that molecular C3D2 desorption is less than 2% of the amount of adsorbed acetylene.
Details
-
File Typepdf
-
Upload Time-
-
Content LanguagesEnglish
-
Upload UserAnonymous/Not logged-in
-
File Pages5 Page
-
File Size-