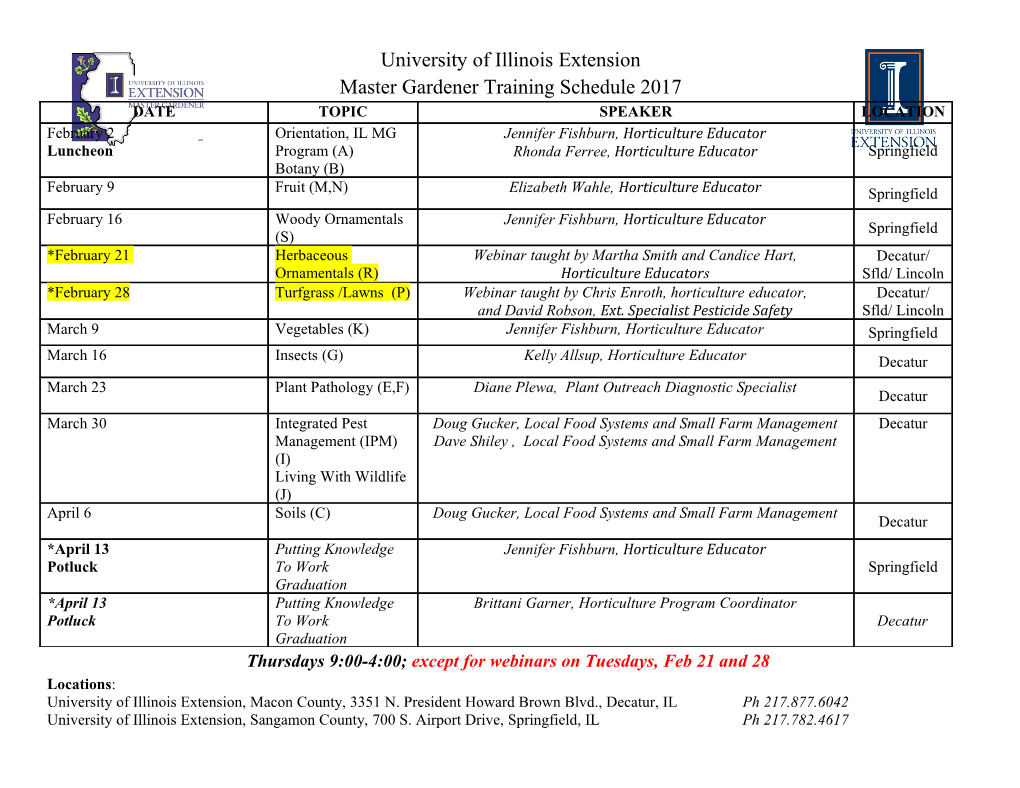
PEPTIDE-BASED SYSTEMS FOR THE TARGETED DISRUPTION AND TREATMENT OF STAPHYLOCOCCUS EPIDERMIDIS BIOFILMS by CHRISTOPHER MICHAEL HOFMANN Submitted in partial fulfillment of the requirements For the degree of Doctor of Philosophy Thesis Advisor: Dr. Roger E. Marchant Department of Biomedical Engineering CASE WESTERN RESERVE UNIVERSITY May, 2012 CASE WESTERN RESERVE UNIVERSITY SCHOOL OF GRADUATE STUDIES We hereby approve the thesis/dissertation of Christopher Michael Hofmann Candidate for the Doctor of Philosophy degree *. (signed) Roger E. Marchant (chair of the committee) James M. Anderson Anirban Sen Gupta Brian Cobb (date) March 29, 2012 *We also certify that written approval has been obtained for any proprietary material contained therein. To my wife and parents, who taught me that anything is possible. TABLE OF CONTENTS TABLE OF CONTENTS 1 LIST OF TABLES 4 LIST OF FIGURES 5 ACKNOWLEDGEMENTS 7 ABSTRACT 8 CHAPTER 1: INTRODUCTION 10 1.1 Clinical Incidence of Infection 10 1.2 Pathogenicity of Implanted Medical Device Infections 11 1.3 Staphylococcus epidermidis Biofilms 13 1.3.1 The Bacteria 13 1.3.2 Primary Adhesion to Surfaces 18 1.3.2.1 Nonspecific Binding 18 1.3.2.2 Bacterial-Mediated Nonspecific Adhesion 23 1.3.2.3 Bacterial-Mediated Specific Binding 24 1.3.2.3.1 Role of Bacterial Polysaccharides as Ligands 25 1.3.2.3.2 Role of Bacterial Proteins as Ligands 26 1.3.3 Biofilm Formation 29 1.3.3.1 Polysaccharide Intercellular Adhesin (PIA) 29 1.3.3.2 Accumulation Associated Protein (AAP) 33 1.3.3.3 Other Matrix Components (eDNA, Bhp, EC TA) 35 1.3.3.4 Quorum Sensing System (Agr, LuxS) 37 1.4 The Host Response 40 1.5 References 45 1 CHAPTER 2: BACTERIAL RESISTANCE TO ANTIBIOTICS 71 2.1 Antibiotics and Resistance to Treatment 71 2.2 Overview of Antibiotics 72 2.3 Antibiotic Resistance 80 2.4 The Biofilm as a Source of Protection 87 2.5 References 98 CHAPTER 3: STRATEGIES FOR PREVENTING AND TREATING 109 DEVICE INFECTIONS 3.1 Modifications of the Biomaterial 109 3.1.1 Surface Modifications of Materials to Prevent Adhesion 109 3.1.2 Incorporation of Antimicrobial Agents 116 3.2 Specific Targeting Strategies 127 3.1.1 Bacteriophages 127 3.1.2 Antibodies and Opsonization 130 3.3 Specific Aims and Hypothesis 133 3.4 References 135 CHAPTER 4: FIBRINOGEN-BASED LIGAND FOR SPECIFIC 143 TARGETING AND DELIVERY TO SURFACE-ADHERENT S. EPIDERMIDIS 4.1 Introduction 143 4.2 Materials and Methods 146 4.3 Results 151 4.4 Discussion 158 4.5 Conclusions 163 4.6 Future Directions 164 4.7 Acknowledgements 167 4.8 References 168 2 CHAPTER 5: DISRUPTION OF S. EPIDERMIDIS BIOFILM 173 FORMATION USING A TARGETED CATIONIC PEPTIDE 5.1 Introduction 173 5.2 Materials and Methods 175 5.3 Results 178 5.4 Discussion 183 5.5 Conclusions 187 5.6 Acknowledgements 188 5.7 References 189 CHAPTER 6: TARGETED DELIVERY OF VANCOMYCIN TO 197 S. EPIDERMIDIS BIOFILMS USING A FIBRINOGEN- DERIVED PEPTIDE 6.1 Introduction 197 6.2 Materials and Methods 200 6.3 Results 207 6.4 Discussion 212 6.5 Conclusions 218 6.6 Acknowledgements 218 6.7 References 219 CHAPTER 7: CONCLUSIONS AND PERSPECTIVES 227 7.1 Summary of Completed Work 227 7.2 Targeted Vancomycin: Synthesis Considerations 229 7.3 Future Directions - Flow System 246 7.4 Acknowledgements 246 7.4 References 250 CHAPTER 8: BIBLIOGRAPHY 253 3 LIST OF TABLES TABLE 2.1 Clinically Administered Antibiotics 73 TABLE 2.2 Modes of Antibiotic Resistance 82 TABLE 6.1 MIC and MBC Values for vancomycin, 208 6-20-PEG3400-VAN, and 6-20-PEG5000-Van 4 LIST OF FIGURES FIGURE 1.1 Schematic of biofilm formation process on 12 cardiovascular biomaterials FIGURE 1.2 Structure of Polysaccharide Intercellular Adhesin (PIA) 32 FIGURE 2.1 Binding interaction between vancomycin and 86 Peptidoglycan Acyl-D-Ala-D-Ala FIGURE 4.1 6-20-NG synthesis scheme 147 FIGURE 4.2 Analysis of 6-20 purity by MALDI-TOF mass 152 spectrometry and RP-HPLC FIGURE 4.3 Blocking non-specific 6-20-NG adhesion to substrate 153 FIGURE 4.4 Normalized 6-20-NG adhesion to PET and S. epidermidis 155 FIGURE 4.5 Scanning electron microscope images of peptide 156 blocking studies FIGURE 4.6 Peptide blocking of 6-20-NG to S. epidermidis 157 FIGURE 5.1 Optical density growth curves of surface-adherent 179 S. epidermidis FIGURE 5.2 Effects of peptide on the composition of surface-adherent 181 biofilms as determined by quantitative fluorescence microplate readings FIGURE 5.3 Biofilm structure after 21 hours as observed by 182 SEM and PIA staining with wheat germ agglutinin FIGURE 5.4 Biofilm composition after 21 hours as determined by the 184 XTT metabolic assay FIGURE 6.1 Chemical structure of vancomycin 202 FIGURE 6.2 Two-step reaction scheme for synthesis of 6-20-PEGX-VAN 203 5 FIGURE 6.3 Retention of 6-20-PEG3400-VAN, 6-20-PEG5000-VAN, 210 and vancomycin by S. epidermidis FIGURE 6.4 Retention of targeted antibiotics by 24 hour 216 S. epidermidis biofilms as determined by indirect ELISA FIGURE 7.1 MALDI-TOF of vancomycin 231 FIGURE 7.2 MALDI-TOF of SM-PEG(12)-Vancomycin synthesized 232 in aqueous conditions (PBS, pH 7.4) FIGURE 7.3 MALDI-TOF of SM-PEG(2)-Vancomycin synthesized 234 in organic conditions (DMF w/ TFA) FIGURE 7.4 MALDI-TOF of SM-PEG(12)-Vancomycin synthesized 235 in organic conditions (DMF w/ TFA) FIGURE 7.5 MALDI-TOF of 6-20-PEG(2)-Vancomycin synthesized 237 in organic conditions (DMF w/ TFA) FIGURE 7.6 pKa values of vancomycin 239 FIGURE 7.7 Charge vs. pH plot for vancomycin and PEGx-Vancomycin 240 FIGURE 7.8 Proposed synthesis scheme for soluble 242 6-20-PEGx-Vancomycin FIGURE 7.9 Proposed synthesis scheme for on-resin modification of 244 6-20 peptide and attachment of MAL-PEGx-NHS crosslinker FIGURE 7.10 MALDI-TOF of MAL-PEG(2)-6-20 synthesized 245 according to scheme in Figure 7.8 6 ACKNOWLEDGEMENTS The Marchant lab has been a wonderful place to learn, and without its many members, past and present, I could never have finished this project. I greatly appreciate the freedom Dr. Marchant allowed me in developing my project, as it allowed me to truly take ownership of the work and its outcome. Former graduate students Sharon Sagnella, Eric Anderson, Arya Kumar, Coby Larsen, and Jeff Beamish were great sources of knowledge and insight as I started out on this journey, while current graduate students Lynn Dudash, Lin Lin, Derek Jones, and Jenny Bastijanic have been great sources of support as I worked to wrap things up. I would especially like to thank Kyle Bednar, who worked with me during his undergraduate time at Case. Without his contributions this work would have taken far longer, and I wish him the best of luck as he now pursues his own Ph.D. at the University of Cincinnati. Most importantly, I want to thank my wife Chrissy for her constant support and encouragement over the years. Her enthusiasm and motivation served as a constant source of inspiration, giving me the strength to keep moving forward even when things weren’t looking so bright. Such an accomplishment would not have been possible without the help and support of countless other people over the years. To those that I wasn’t able to thank by name, please know that I will be forever grateful for everything you have done on my behalf. 7 Peptide-Based Systems for the Targeted Disruption and Treatment of Staphylococcus epidermidis Biofilms Abstract by CHRISTOPHER MICHAEL HOFMANN Complications due to nosocomial infections of implanted medical devices pose a significant health risk to patients, with Staphylococcus epidermidis often implicated in the case of blood-contacting biomaterials. One method by which S. epidermidis initially adheres to biomaterials uses the plasma protein fibrinogen as an intermediary, where the S. epidermidis surface protein SdrG binds to a short amino acid sequence near the amino terminus of the B chain of fibrinogen. This study reports on the use of this fibrinogen-derived 6-20 peptide for the targeted disruption of S. epidermidis biofilm formation using a cationic peptide, as well as the specific delivery of vancomycin to S. epidermidis biofilms. S. epidermidis virulence relies mainly upon its ability to form a biofilm, the main component of which is polysaccharide intercellular adhesin (PIA). The synthetic 6-20 peptide was utilized to deliver a cationic polylysine peptide (G3K6) to the bacterial surface and disrupt the charge-charge interactions needed for PIA retention and biofilm stability. The effects of the 6-20-G3K6 peptide on biofilm formation were assessed using optical density, fluorescently labeled wheat germ 8 agglutinin, nucleic acid stain (SYTO 9), and a metabolic assay (XTT, 2,3-bis(2- methoxy-4-nitro-5-sulfophenyl)-2H-tetrazolium-5-carboxanilide inner salt). Biofilms formed in the presence of 6-20-G3K6 peptide (100 M) resulted in a 37.9% reduction in PIA content and a 17.5% reduction of adherent bacteria relative to biofilms grown in the absence of peptide. These studies demonstrate the targeting ability of the 6-20 peptide towards biomaterial-adherent S. epidermidis, and highlight the potential for disrupting the early stages of biofilm formation. Targeted 6-20-PEGx-VAN vancomycin derivatives were then synthesized using a flexible, variable length poly(ethylene glycol) linker between the peptide and antibiotic. Initial binding to surface adherent S. epidermidis was increased in a concentration-dependent manner relative to vancomycin for all equivalent concentrations ≥4 g/ml, with targeted vancomycin content up to 22.9 times that of vancomycin alone.
Details
-
File Typepdf
-
Upload Time-
-
Content LanguagesEnglish
-
Upload UserAnonymous/Not logged-in
-
File Pages300 Page
-
File Size-