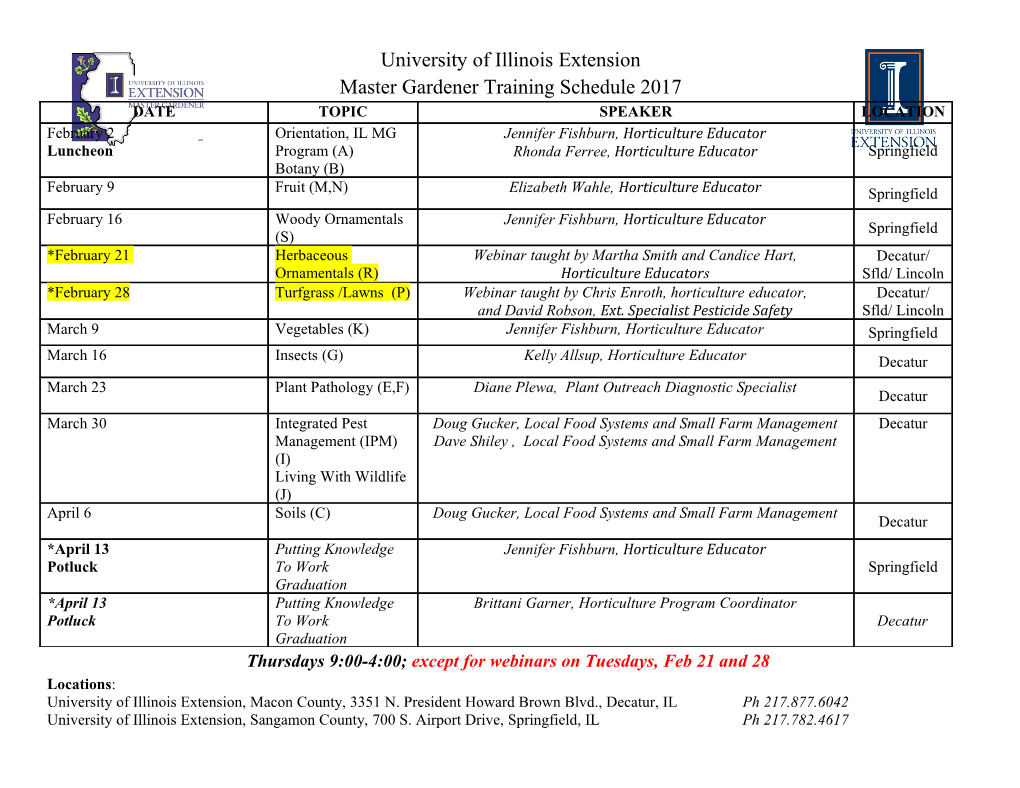
Opinion Beyond DNA origami: the unfolding prospects of nucleic acid nanotechnology Nicole Michelotti,1 Alexander Johnson-Buck,2 Anthony J. Manzo,2 and Nils G. Walter2,∗ Nucleic acid nanotechnology exploits the programmable molecular recognition properties of natural and synthetic nucleic acids to assemble structures with nanometer-scale precision. In 2006, DNA origami transformed the field by providing a versatile platform for self-assembly of arbitrary shapes from one long DNA strand held in place by hundreds of short, site-specific (spatially addressable) DNA ‘staples’. This revolutionary approach has led to the creation of a multitude of two-dimensional and three-dimensional scaffolds that form the basis for functional nanodevices. Not limited to nucleic acids, these nanodevices can incorporate other structural and functional materials, such as proteins and nanoparticles, making them broadly useful for current and future applications in emerging fields such as nanomedicine, nanoelectronics, and alternative energy. 2011 Wiley Periodicals, Inc. How to cite this article: WIREs Nanomed Nanobiotechnol 2012, 4:139–152. doi: 10.1002/wnan.170 INTRODUCTION Inspired by nature, researchers over the past four decades have explored nucleic acids as convenient ucleic acid nanotechnology has been utilized building blocks to assemble novel nanodevices.1,10 by nature for billions of years.1,2 DNA in N Because they are composed of only four different particular is chemically inert enough to reliably chemical building blocks and follow relatively store genetic information over even millions of simple, yet highly specific and thus predictable years.3 Packaged in genomes, DNA is expressed organizational base pairing rules at the molecular in a regulated fashion with assistance from its scale, nucleic acids are the preferred biological more rapidly hydrolyzed structural analog, RNA, material for the design of structures with nanometer as well as protein factors.2,4,5 Complementarily, the precision when compared with other candidates enhanced functionality of RNA is exploited in many such as proteins. Short [typically <50 nucleotides biological nanomachines, such as the ribosome6 (nt) for RNA and <100 nt for DNA] specific and the spliceosome,7,8 for the ‘data processing’ sequences of the building blocks can be chemically that accompanies gene expression. Nature also uses synthesized at low cost, whereas longer DNA strands the specificity of base pairing by nucleic acids of predefined sequence are provided by nature for database maintenance and readout via the in the form of genomic DNA. A combination modulation of gene expression by noncoding RNAs of these two sources led to the advent of the (ncRNAs).4,9 DNA origami method in 2006,11 which dramatically accelerated progress in nucleic acid nanotechnology ∗ by further increasing the simplicity, precision, Correspondence to: [email protected] and fidelity of the design principles available for 1Department of Physics, University of Michigan, Ann Arbor, MI, USA generating spatially addressable nanoscale structures 2Department of Chemistry, University of Michigan, Ann Arbor, (Box 1). Numerous DNA origami-based nucleic MI, USA acid nanodevices and nanomaterials have since been Volume 4, March/April 2012 2011 Wiley Periodicals, Inc. 139 Opinion wires.wiley.com/nanomed constructed12–16 with great potential for a multitude THE BUILDING MATERIALS: of useful applications and with abundant prospects NATURAL NUCLEIC ACIDS for innovation. Nucleic acids are linear biopolymers found in all organisms, as well as many viruses, as they are the means by which genetic information is stored, BOX 1: DNA ORIGAMI transferred, and regulated. Their monomeric building blocks (nucleotides) each consist of three moieties The invention of scaffolded DNA origami (Figure 1(a)–(c)): a nucleobase, a five-carbon sugar, was a milestone in the advancement of and a phosphate group. The phosphate group nucleic acid nanotechnology. Developed by Paul enables the formation of a phosphodiester bond Rothemund, DNA origami is the assisted folding between the sugars of adjacent nucleotides, creating of one long ssDNA ‘scaffold’ strand from a a polymer known as a single-stranded nucleic acid. bacterial phage genome consisting of 7249 Complementary nucleobases can form hydrogen nucleotides into a predetermined shape by bonds which, along with stacking interactions ∼200 typically 32-nt-long ssDNA ‘staple’ strands containing sequences that are complementary between adjacent base pairs, result in two fully to specific regions of the scaffold strand. complementary polymers hybridizing to form a 17 Two or more nonadjacent segments of the relatively rigid antiparallel double-stranded helix scaffold strand are brought together and (Figure 1(d)). This famous Watson–Crick duplex held in place by hybridization to different has an average dimension (rise) of 0.34 nm portions of the same staple oligonucleotide in (for canonical B-form DNA) between the base aggregate (Figure 3(a)), enabling the creation pairs along the helical axis and a diameter of of arbitrary shapes based solely on the staple 2 nm, ideally suited to engineer nanotechnological sequences (Figure 3(b) and (c)). The staples may structures. be extended at their 5 ends to make them Nature has optimized two different types of addressable for patterning with a resolution nucleic acids through evolution: DNA and RNA, of 6 nm on the assembled origami, imposed by which both consist of four nucleotide building blocks the interstaple distance on the assembled DNA that tend to pair in a predictable Watson–Crick 11 duplexes of the origami (Figure 3(d)). fashion (Figure 1(d)). The guanine–cytosine (G:C) While building on previous approaches base pair forms three hydrogen bonds, stronger than to self-assembly of DNA nanostructures, the the two hydrogen bonds of the adenosine–thymine DNA origami technique has multiple advan- (A:T) pair, used in DNA, or the adenosine–uracil tages: (1) It accomplishes highly specific topolo- (A:U) pair, used in RNA. The primary chemical gies with a spatially addressable resolution difference between DNA and RNA is their comparable to that accomplished by surface sugars (Figure 1(a)); ribose, the sugar found in manipulation using atomic force microscopy RNA, contains an extra 2-hydroxyl group not (AFM) or scanning tunneling nicroscopy (STM). (2) The well-formed, redundant self-assembly is present in deoxyribose, the sugar found in DNA, ∼ relatively insensitive to varying stoichiometric causing the backbone of RNA to hydrolyze 8 10 ratios of the staples, eliminating the need for 10 -to10 times more quickly than that of 18 intermittent purification steps and resulting in DNA (Figure 1(a)). The A-form RNA duplex, higher yields. (3) Multiple nanostructures may however, is more thermally stable than the B-form be obtained simultaneously and possibly fur- DNA duplex, even though RNA is predominantly ther assembled into larger structures with high found single-stranded in nature and forms mostly fidelity. (4) Creating arbitrary shapes that are intramolecular helices.19 These structural differences largely unrestricted by symmetry considerations between DNA and RNA are functionally exploited by is relatively straightforward.11 nature. The DNA origami method has so far provided a scaffold for many applications including forming the track for molecular DNA nanorobots,47 constructing ordered molecular assembly lines,48,49 and assembling components While the most notable purpose of DNA is storing for nanoelectronic circuits.50,51 The scope and and maintaining the genetic blueprint of an organ- limitations of these applications are enumerated ism, DNA performs other structural roles in the throughout. cell as well. For instance, the guanine-rich single- stranded telomeres at the ends of chromosomes can 140 2011 Wiley Periodicals, Inc. Volume 4, March/April 2012 WIREs Nanomedicine and Nanobiotechnology Beyond DNA origami (a) (d) DNA/RNA 3' R = CH3 (thymine), O O 5' Base H (uracil) Base O H O O H H O R N NH2 O H H O N N HN O B H H N N O O OH O H OH, OCH3, H H NH , F O H O 2 -O O A T/U -S-O P O P - O P O- O P O O O AH+ O H O H H N O H2N H O N NH N H H H N N O NH O H H 2 (b)PNA (c) LNA O O H G C - O P O- O P O HN O Base O H O Base O H H O H2N NO2 N H H O N N HN O O H H N NH O N O 2 O H H O H O PZ -O PO 5' NH O 3' O FIGURE 1| Chemical structures of nucleic acids. Nucleotide backbone structures for (a) DNA (left), RNA (right), (b) PNA, and (c) LNA. The right side of panel (a) also indicates the backbone hydrolysis reaction of RNA, where ‘AH+’ and ‘B’ are an acid and base catalyst, respectively. (d) Structure of a double-stranded nucleic acid incorporating the four principal natural nucleobases A, T/U, G, and C, as well as the artificial P:Z base pair. form topologically more complex quadruplex struc- importance of RNA that does not code for proteins tures built around square stacks of four guanine bases have been increasingly appreciated. Typically, these stabilized by a chelated metal ion (preferably K+) ncRNAs directly or indirectly regulate or mediate at their center. G-quadruplexes thus play important gene expression at the transcriptional or transla- roles in the maintenance of linear eukaryotic genomes tional level, making them candidates for therapeutic and possibly in gene expression regulation.20 Simi- applications.4,9,26 Some ncRNAs simply make use of larly, so-called Holliday four-way junctions of two molecular recognition through base pairing, such as entwined DNA duplexes play critical roles in DNA in the RNA interference pathway.26 Others exploit recombination.21 Forming and resolving such higher- conformational dynamics of RNA.27 For example, order structures often requires the aid of external riboswitches regulate gene expression via the confor- factors such as proteins.
Details
-
File Typepdf
-
Upload Time-
-
Content LanguagesEnglish
-
Upload UserAnonymous/Not logged-in
-
File Pages14 Page
-
File Size-