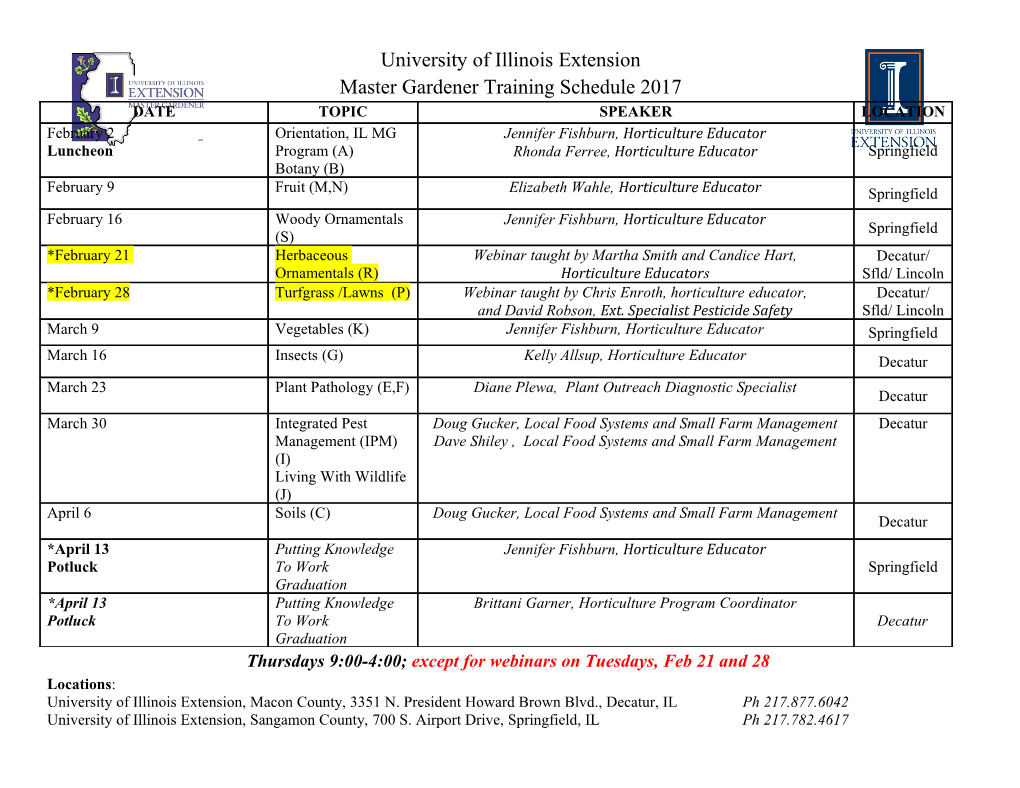
Evolution off the Main Sequence Zero Age Main Sequence (ZAMS) stars are defined as being chemically homogeneous. However, because (most) stars are not fully convective, nuclear fusion in the stellar cores will create chemical gradients. In particular, the fraction of hydrogen in the cores will decrease, as hydrogen is fused into helium. When the hydrogen fraction in the center of the star declines to ~5%, the main sequence phase ends. Evolution to the Giant Branch Roughly speaking, a star’s main sequence hydrogen-to-helium burning core consists of the inner ~10% (by mass) of the star. When all the core hydrogen is used up • The core begins to collapse, as there is no longer an energy source to maintain its central pressure. The core’s gravitational potential energy is converted into thermal energy. • The core collapse also increases the pressure just outside the core, causing hydrogen fusion in a thick shell around the core (~5% of the star). This shell will eventually get very thin (~ 0.5% by mass). • As conditions in the shell become more extreme, fusion proceeds via CNO burning, with the rate entirely a function of the core mass, i.e., L µ Mg, with g ~ 8. • The radiation pressure associated with shell burning pushes matter away in both directions. • Quick rule of thumb: shells inverse expansion. If the core is contracting, the material outside a shell is expanding. The post main-sequence star has 2 energy sources: the gravitational contraction of the core, and the hydrogen fusion occurring in the shell around the core. The amount of material fusing is small, but the rate of fusion is high. During the giant branch, the evolutionary rate constantly increases: shell fusion produces helium which becomes part of the core, which increases the mass of the core, which increases the rate of fusion. Evolution to the Giant Branch • As the shell narrows, the star adjusts its structure on a thermal timescale. As the area outside the shell is pushed farther away by radiation pressure, it begins to cool. The area becomes more opaque because of Kramer’s opacity law (κ µ T-3.5). • The higher opacity of the envelope traps the energy, which does PdV work on its surroundings, causing the gas to expand. This further cools the gas, which increases the opacity, which traps more energy, which causes further expansion. The star crosses the “Hertzprung Gap” (the region between the main sequence and the red giant branch in the HR diagram), with the expansion driven mostly by its own thermal energy. • As the outside of the star cools, conditions become more conducive to convection (again, since κ µ T-3.5). The convective envelope reaches deeper and deeper into the star. Eventually, the star is almost fully convective (except for the inert core and the very thin hydrogen burning shell). Evolution on the Giant Branch • During their main sequence phase, high-mass stars have cores that become smaller with time. When the stars become giants, their convective envelope can reach the outer region of the early-main sequence core, and “dredge-up” some CNO-processed material. • Eventually, the star reaches the “Hayashi line.” (Energy-producing stars cannot be cooler than this line.) • Kramer’s-style opacity dominates throughout most of the star, but at the surface of cool stars, H- is the dominant source of opacity. The requisite electrons (for bound free and H-) come from metals; consequently, the lower the star’s metal abundance, the lower the opacity, the less energy is trapped, the less PdV work is done, the smaller the stellar expansion, and the hotter the star. The location of the Hayashi line is temperature dependent: metal-poor giants are bluer than metal-rich giants. Evolution of the Central Conditions The Triple-a Process • Helium is inert because 8Be exists for only ~ 10-16 seconds. However, eventually, the core becomes dense enough so that the reaction 4He + 4He D 8Be + 4He g 12C* becomes possible. Unfortunately, the decay of excited carbon, 12C*g12C is highly unlikely; much more likely is 12C* g 4He + 8Be. Still, once the 4He + 4He g 8Be + 4He g 12C* g12C reaction starts, its energy 40 quickly causes more reactions, creating a runaway, with ε3αµT . This is called the triple-α process. • Due to electron degeneracy, stars with M < 2.3 M8 all ignite helium at a core mass of ~ 0.46 M8. At this time, the star’s luminosity (to within a few percent) is ~ 2500 L8 • With triple-α also comes the reaction 12C + 4He g 16O. But the resonance for this reaction is not well-known, so the resulting ratio of 12C/16O can be almost anything. (Consequently, it is usually assumed to be 50%-50%.) Although the electrostatic repulsion is greater, the 16O + 4He g 20Ne reaction also occurs. The Helium Flash • When helium ignites in low mass stars (M < 2.3 M8), it does so degenerately in a thermonuclear runaway called the “Helium Flash”. 11 At maximum, the luminosity from this fusion is 10 L8, like a supernovae! However, almost none of this energy reaches the surface; it all goes into lifting the core degeneracy and then heating up the star. As a result, the core expands, hydrogen shell burning ceases (or becomes very small), the stellar opacity declines, less heat is trapped, and the star becomes smaller and fainter. • In higher mass stars, helium ignites non-degenerately. In very high mass stars (M > 15 M8), it may even ignite before the star gets to the giant branch. (In these stars, the nuclear timescale is similar to the thermal timescale.) Mass Loss • On the giant branch (and especially on the return to the giant branch), mass loss becomes important. A “reasonable” mass-loss law is L R M˙ ∝ M (In other words, the mass lost from a star is proportional to the luminosity blowing the material away, and inversely proportional to the gravitational€potential at the stellar surface.) The constant of proportionality for the equation is defined by the Sun, which loses -13 roughly 4 × 10 M8/yr (the solar wind). This is the Reimers’ mass- loss law. It is almost certainly wrong, but in some cases, it is perhaps not too far wrong. During the red giant branch, mass loss occurs, but is relatively small; in total, about ~ 0.2 M8 is lost. Stellar Sizes on the Red Giant Branch • The maximum size a star attains before igniting helium depends on its mass. Electron degeneracy helps support the core of lower masses stars, delaying the ignition. As a result, lower mass stars get larger than higher mass stars. Horizontal Branch Stars • After helium ignition, helium fusion occurs in the center of the (previously inert) helium core. Hydrogen continues to fuse in a shell outside the core. Horizontal Branch Stars • low mass stars (M ≲ 1 M8) which ignite helium degenerately settle onto the “Horizontal branch,” with luminosities of ~ 75 L8. The temperature of these stars depend on their envelope mass: the more matter on top of the hydrogen burning shell, the cooler the star. Horizontal Branch Stars • low mass stars (M ≲ 1 M8) which ignite helium degenerately settle onto the “Horizontal branch,” with luminosities of ~ 75 L8. The temperature of these stars depend on their envelope mass: the more matter on top of the hydrogen burning shell, the cooler the star. • The bluest horizontal branch stars have masses ~ 0.5 M8; the reddest, ~ 1 M8. • Some horizontal stars have very small envelopes (~ 0.05 M8), and thus extremely blue colors. These are sometimes called extreme horizontal branch stars (EHB); they appear on the “vertical part of the horizontal branch”. • Helium fusion is much less efficient than hydrogen fusion, so the horizontal branch phase is relatively short (~ 100 Myr). When helium in the core runs out, the core again contracts, and a thick helium shell-burning stage begins. The star starts its trip to the Asymptotic Giant Branch (AGB). Blue Loop Stars Higher mass stars burn helium in their core non- degenerately; their luminosity is a function of mass, and they evolve through a “blue loop” phase. Early Asymptotic Giant Branch Stars • During its early AGB phase, a star has an inert C/O core, a thick helium burning shell, a hydrogen burning shell, and an envelope that will eventually extend to over 1 A.U. • The evolution of AGB stars parallels that of RGB stars, with 3 sources of energy: the contracting core, the helium burning shell, and the hydrogen burning shell. The energy for expanding the star comes mostly from the heat of the star itself, through the behavior of Kramers opacity. Thermal Pulse Asymptotic Giant Branch Stars • Helium fusion in a thin shell is extremely unstable. When helium ignites in a thin shell, the energy expands the region around it and extinguishes the hydrogen burning shell. After a while, helium shell burning ceases, and the hydrogen shell-burning starts again. These “Thermal Pulses” occur on timescales between 105 years and 10 years, depending on the mass of the core. Thermal Pulse Asymptotic Giant Branch Stars • Helium fusion in a thin shell is extremely unstable. When helium ignites in a thin shell, the energy expands the region around it and extinguishes the hydrogen burning shell. After a while, helium shell burning ceases, and the hydrogen shell-burning starts again. These “Thermal Pulses” occur on timescales between 105 years and 10 years, depending on the mass of the core. • Because of the thermal pulses, mass loss is greatly enhanced -4 during the TP-AGB phase, and may reach ~ 10 M8/yr. This is called a “superwind,” which comes off the star at ~ 10 to 20 km/s.
Details
-
File Typepdf
-
Upload Time-
-
Content LanguagesEnglish
-
Upload UserAnonymous/Not logged-in
-
File Pages50 Page
-
File Size-