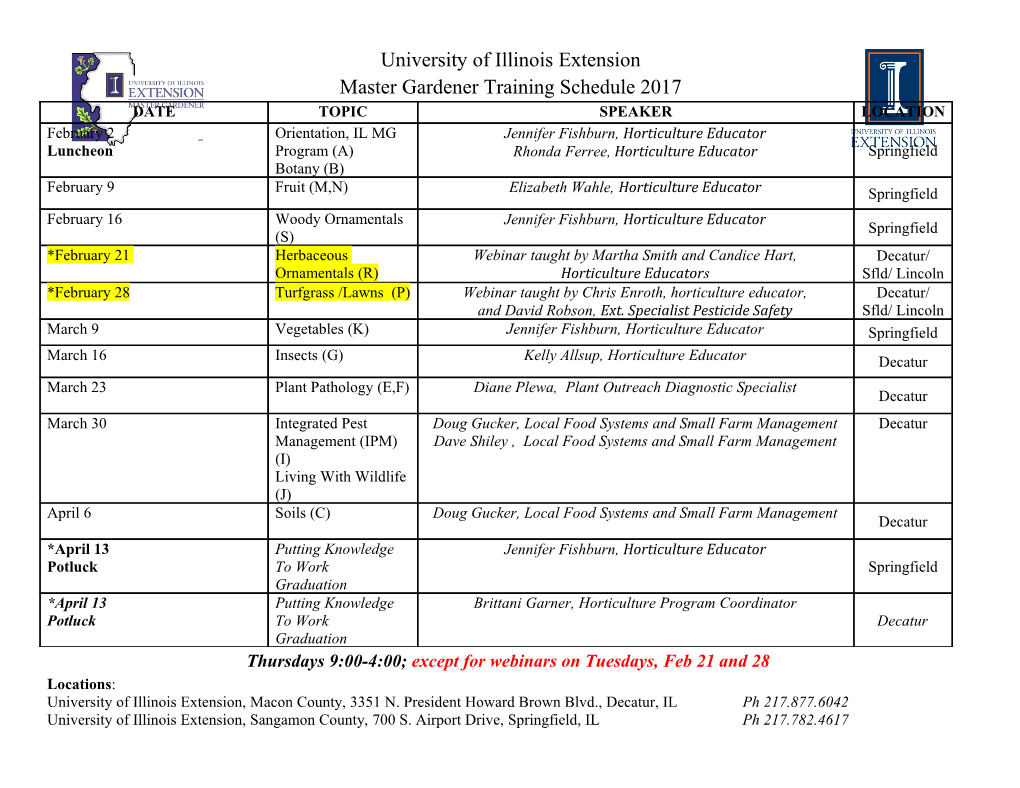
Oncogene (2014) 33, 3351–3360 & 2014 Macmillan Publishers Limited All rights reserved 0950-9232/14 www.nature.com/onc REVIEW ATM signalling and cancer CA Cremona and A Behrens ATM, the protein kinase mutated in the rare human disease ataxia telangiectasia (A-T), has been the focus of intense scrutiny over the past two decades. Initially this was because of the unusual radiosensitive phenotype of cells from A-T patients, and latterly because investigating ATM signalling has yielded valuable insights into the DNA damage response, redox signalling and cancer. With the recent explosion in genomic data, ATM alterations have been revealed both in the germline as a predisposing factor for cancer and as somatic changes in tumours themselves. Here we review these findings, as well as advances in the understanding of ATM signalling mechanisms in cancer and ATM inhibition as a strategy for cancer treatment. Oncogene (2014) 33, 3351–3360; doi:10.1038/onc.2013.275; published online 15 July 2013 Keywords: ATM; signalling; cancer INTRODUCTION TRRAP proteins) and FATC domains at the C terminus, the protein ATM, the gene mutated in ataxia telangiectasia (A-T), has been also contains N-terminal HEAT repeats (named after the proteins associated with cancer since its discovery, because A-T patients huntingtin, elongation factor 3, the A subunit of PP2A and TOR1) are prone to thymic lymphoma and other cancers.1 The first and a substrate-binding domain. The kinase domain is responsible explanation for this predisposition was that ATM encodes a kinase for several autophosphorylations, as well as phosphorylating the 3 central to the repair of double-strand breaks (DSBs) in DNA, hundreds of ATM substrates identified so far. The high number of thereby controlling genome stability and cell survival (reviewed in targets and extensive potential for modulation places ATM as a Shiloh2). A-T cells show radioresistant DNA synthesis—failure to signalling hub, with many different inputs and a complex output stop or slow cell-cycle progression in response to radiation subject to the actions of cofactors, substrates and feedback damage—revealing a lack of checkpoint control that clearly regulation. contributes to cancer predisposition. However, this is just one aspect of ATM’s function. Over recent years, a wealth of evidence has accumulated showing that ATM is part of many other ATM SIGNALLING signalling networks, including cell metabolism and growth, ATM signalling can be broadly divided into two categories: a oxidative stress, and chromatin remodelling, all of which can canonical pathway, which signals together with the Mre11-Rad50- affect cancer progression. These diverse effects mean that the NBS1 (MRN) complex from DSBs and activates the DNA damage predisposition to cancer of various types, and its clinical course in checkpoint, and several non-canonical modes of activation, which each case, is altered by inherited and spontaneous ATM mutations. are activated by other forms of cellular stress (Figure 2). Both Although ATM is rightly considered to be a tumour suppressor, signalling categories are likely to contribute to ATM’s role in ATM signalling can also be advantageous to cancer cells, tumour suppression. particularly in resistance to radio- and chemotherapeutic treat- ment. For this reason, ATM inhibitors have been developed for use Canonical ATM signalling at DSBs in cancer therapy. Here we will review these apparently ATM kinase is present in its inactive form as a non-covalently paradoxical ATM functions with reference to ATM signalling in 4 cancer cell and mouse models as well as human patients. linked dimer. Canonical ATM activation, in response to DSBs, involves dimer dissociation, activation of monomers and recruitment to DNA break sites. The MRN complex is required ATM—a multifunctional kinase for ATM recruitment to DSBs and efficient activation: the NBS1 The ATM protein is a very large, 370 kDa kinase encoded on subunit interacts directly with ATM, and ubiquitination of NBS1 human chromosome 11q22-23. Mouse ATM, encoded on chromo- helps ATM recruitment.5–7 The end clipping activity of MRN also some 9, is 84% identical to the human protein, and homologs contributes to ATM activation by generating short ssDNA oligos have been found in all eukaryotes. Because of its size, a detailed that associate with it.8 Ionising radiation (IR)-induced ATM atomic structure of the whole protein has not yet been activation via MRN results in the phosphorylation of a vast determined, but a combination of extensive mapping of patient network of substrates, including the key checkpoint kinase Chk2 mutations, in vitro studies and comparison with related phospha- and the tumour suppressor p53 (Figure 2). tidylinositol-like superfamily proteins has resulted in a well- ATM autophosphorylation at serine 1981 (Ser1981; equivalent defined domain structure incorporating many post-translational to Ser1987 in mice) occurs within 1 min of irradiation and is widely modification sites (Figure 1). As well as a PI3K-like serine/threonine used as a read-out of ATM activation.4,9 However, in the absence kinase, which is flanked by FAT (named after the FRAP, ATR and of MRN, ATM monomers are not phosphorylated at Ser1981 but Mammalian Genetics Lab, Cancer Research UK London Research Institute, London, UK. Correspondence: Dr A Behrens, Mammalian Genetics Lab, Cancer Research UK London Research Institute, 44 Lincoln’s Inn Fields, London WC2A 3LY, UK. E-mail: [email protected] Received 26 March 2013; revised 17 May 2013; accepted 20 May 2013; published online 15 July 2013 ATM and cancer CA Cremona and A Behrens 3352 HEAT repeats P P P P P Cys P Chromatin binding Substrate binding NLS Caspase 3 sites Leucine zipper NBS1 binding FAT PI3K FATC ATM N C 3056 a.a. 367 794 1403 1893 1981 2996 Figure 1. Domain structure of human ATM. ATM can be divided into an N-terminal half that is largely unique, and a C-terminal half that has homology to other phosphoinositide-3 kinase (PI3K)-like kinases such as ATR, mTOR and DNA-PKcs. This conserved portion contains a FAT domain (named after the FRAP, ATR, and TRRAP proteins), the PI3K-like serine/threonine kinase domain (here shown in red), and a FAT C-terminal domain (FATC). ATM contains at least five autophosphorylation sites: serines 367, 1893, 1981 and 2996, whose phosphorylation is induced after IR; and threonine 1885, which is not induced by IR.189 Cdk5 phosphorylates serine 794 in postmitotic neurons,190 and Aurora B phosphorylates serine 1403 in mitosis.191 In oxidising conditions, cysteine 2991 forms a disulphide linkage with a second ATM molecule to form a dimer.68 The N-terminal portion interacts with substrates and cofactors such as NBS1, p53, BRCA1, LKB1 and BLM; amino acids 91–97 (here shown in purple) are required for IR-induced formation of nuclear ATM foci.192 Also in the N-terminal portion are a proposed chromatin- interaction domain, a nuclear localisation sequence (NLS), two caspase 3 cleavage sites, and a putative leucine zipper region.193–195 The majority of the protein is composed of 49 HEAT repeats (named after the proteins huntingtin, elongation factor 3, the A subunit of PP2A and TOR1).196 These likely mean that ATM is highly flexible; indeed, a low-resolution structural study of ATM found a flexible arm region that changes conformation upon DNA binding.197 Diagram produced using Domain Graph 2.0.198 17–19 Canonical Non-canonical Redox damage sites. RNF168 monoubiquitylates histones H2A and Ionising Chromatin changes H2AX on lysines 13 and 15 and primes them for polyubiquity- radiation (hypotonic stress; chloroquine) Oxidation lation, necessary for recruitment of further repair factors.20 ATM and Mdc1 determine the spreading of gH2AX along chromatin, while ubiquitin enzymes control spreading of ubiquitylated histones.21,22 Continued ATM activation is required to maintain ATMATM ATM ATM ATM ATM damage foci even when ubiquitylated regions are expanded.22 A complex feedback system dynamically tunes ATM signalling to the existing damage—as long as damage persists above a threshold, ATM is activated in a pulse-like manner due to feedback 23 P P P P from activated p53. The molecular details of ATM signalling 24–26 ATM NBS1 ATM ATMIN ATM ATM at DSBs have been extensively reviewed. Here we will highlight the emerging interplay between ATM and chromatin in this response. Many groups have shown that ATM is important for the repair of DSBs at diverse genome locations, including euchromatic P P P P P 15,27,28 Chk2 Chk2 Chk2 Smc1 Smc1 immunoglobulin loci, while others have found that it is particularly required for DSBs located in heterochromatin.29,30 P P P Kap1 Kap1 ? Although experiments with laser lines show a local P P P 9 p53 p53 p53 decondensation of chromatin at DSBs that is ATM-independent, P ATM also decondenses chromatin to increase accessibility to ? • DSB repair repair machinery and reinforce its own activation. ATM-dependent • Cell cycle arrest • Genome stability phosphorylation of RNF20/RNF40 leads to ubiquitination of • Radiosensitivity • Control of cell survival • Oxidative stress response histone H2B, and ATM phosphorylation of Kap1 reduces its affinity for the chromodomain protein CHD3, both of which lead Figure 2. Canonical and non-canonical ATM signalling pathways. 31–33 ATM signals together with the MRN complex at DSBs (canonical to decompaction of chromatin structure. pathway, left); together with ATMIN following non-DSB stimuli such Chromatin relaxation at DSB sites also ensures efficient ATM as oxidative stress and chromatin changes (middle) and can also activation. It has long been known that histone deacetylase form disulphide dimers in oxidative stress conditions (right). inhibitors activate ATM,4 and Tip60 mediates acetylation of Chromatin remodelling around DSBs also contributes to canonical histones H4 and H2AX to remodel chromatin at DSB sites.34 ATM activation.
Details
-
File Typepdf
-
Upload Time-
-
Content LanguagesEnglish
-
Upload UserAnonymous/Not logged-in
-
File Pages10 Page
-
File Size-