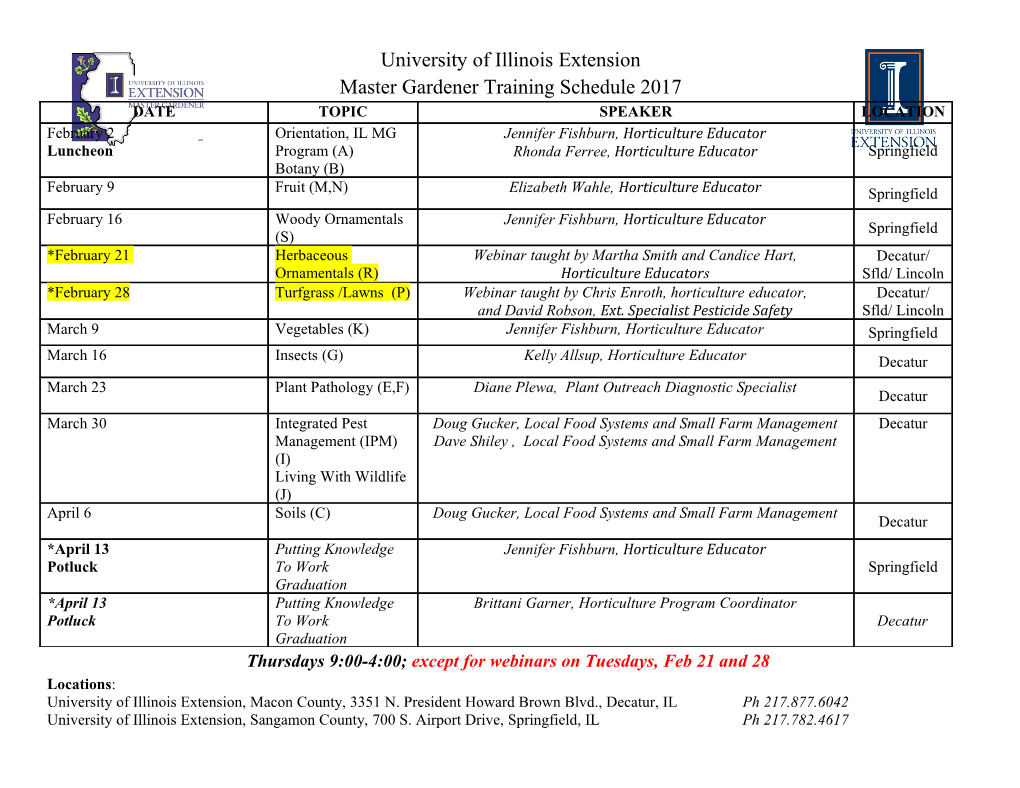
LASER-INDUCED ACOUSTIC DESORPTION ATMOSPHERIC PRESSURE PHOTOIONIZATION MASS SPECTROMETRY FOR COMPLEX MIXTURE ANALYSIS A Dissertation Presented to The Academic Faculty by Kevin Andrew Benham In Partial Fulfillment of the Requirements for the Degree Doctorate of Philosophy in the School of Chemistry and Biochemistry Georgia Institute of Technology May 2019 COPYRIGHT © 2019 BY KEVIN BENHAM LASER-INDUCED ACOUSTIC DESORPTION ATMOSPHERIC PRESSURE PHOTOIONIZATION MASS SPECTROMETRY FOR COMPLEX MIXTURE ANALYSIS Approved by: Dr. Thomas Orlando, Advisor Dr. Ronghu Wu School of Chemistry and Biochemistry School of Chemistry and Biochemistry Georgia Institute of Technology Georgia Institute of Technology Dr. Facundo Fernandez Dr. Faisal Alamgir School of Chemistry and Biochemistry School of Materials Science and Georgia Institute of Technology Engineering Georgia Institute of Technology Dr. Amanda Stockton School of Chemistry and Biochemistry Georgia Institute of Technology Date Approved: February 27th , 2019 Seek the truth, always question, and be ever vigilant of truth’s many imposters. ACKNOWLEDGEMENTS Beginning with the agencies responsible for supporting this work, I must first acknowledge the Center for Chemical Evolution at the Georgia Institute of Technology for their continued and unwavering support of this research. Additionally, this work acknowledges the parent organizations, the National Science Foundation (NSF) and National Aeronautics and Space Administration (NASA) for bestowing the Georgia Institute of Technology with this prestigious research center. On an individual basis, I’d first like to make mention of the difficult and pioneering work of my predecessor, Dr. Joshua Symonds and for leaving me with a feasible and promising body of microplasma work to build upon. Other notable mentions of colleagues in my time in the EPICS lab include Dr. Reuben Gann for providing myself and others with extensive practical lab knowledge and the example he set as an inquisitive and thorough scientist. I must also thank my close classmates and colleagues, Aaron McKee, Alex Elder, Brant Jones, Micah Schaible, and Alma Castaneda for their support throughout this journey. And of course, I thank my advisor Professor Thomas Orlando for giving me the freedom and space to pursue my curiosities and interests, even when they may have been tangential to this research. Aside from the EPICS lab I would also like to thank the members of the Fernández lab for their help on getting started with the complex instrumentation employed throughout this work. I also wish to thank Professor Facundo Fernández for the significant amount of resources provided through his lab to reach the accomplishments made by this research. iv The progress afforded through the kind gifts of instrument time and usage was crucial to the success of this work. Lastly, I would like to thank my fiancé Elyse Paske, and my family. Your support, kindness, and encouragement have been indispensable throughout this odyssey. v TABLE OF CONTENTS ACKNOWLEDGEMENTS iv LIST OF TABLES ix LIST OF FIGURES x LIST OF SYMBOLS AND ABBREVIATIONS xiv SUMMARY xvi CHAPTER 1. INTRODUCTION 1 Context and Motivation 1 Ambient and Atmospheric Ionization Sources 2 1.2.1 Atmospheric Pressure Ionization Sources 2 1.2.2 Ambient Ionization Sources 8 Technologies of LIAD-APPI 12 1.3.1 LIAD 12 1.3.2 Plasma fundamentals and microplasmas 15 Summary 22 CHAPTER 2. LASER-INDUCED ACOUSTIC DESORPTION ATMOSPHERIC PRESSURE PHOTOIONIZATION VIA VUV-GENERATING MICROPLASMAS 23 Introduction 23 Experimental 26 2.2.1 Materials, Chemicals, and Sample Preparation 26 2.2.2 Microplasma Device 26 2.2.3 Microplasma Operation 28 2.2.4 VUV Monochromator Instrumentation 29 2.2.5 LIAD-APPI Setup 29 Results and Discussion 30 2.3.1 Microplasma Lyman-α Output 31 2.3.2 LIAD-APPI of Low-Polarity Organics 32 2.3.3 Mechanisms of LIAD-APPI and Detection Limits 35 Conclusion 38 Acknowledgements 39 CHAPTER 3. SWEEP JET COLLECTION LASER INDUCED ACOUSTIC DESORPTION ATMOSPHERIC PRESSURE PHOTOIONIZATION FOR LIPID ANALYSIS APPLICATIONS 41 Introduction 41 Experimental 44 3.2.1 Materials and Chemicals 44 3.2.2 Sample Preparation & Deposition 45 vi 3.2.3 MHCD Device & Operation 46 3.2.4 Sweep Jet LIAD-APPI Setup 48 3.2.5 Mass Spectrometry Experiments 49 3.2.6 CFD Simulations and Computational Details 49 Results and Discussion 50 3.3.1 Sweep Jet Collection Improvements 50 3.3.2 CFD and Particle Trajectory Simulations 53 3.3.3 Sterols and Sterol Esters 58 3.3.4 Triacylglycerols 62 3.3.5 Towards Complex Mixtures - EVOO and Chloroform Extracts of Australian Shale 64 3.3.6 LIAD Mechanism - Oil desorption signal response 68 Conclusion 69 Acknowledgments 70 Supplemental Sphingomyelin Results 71 CHAPTER 4. ANALYSIS OF CRUDE OIL AND SHALE ROCK HYDROCARBONS VIA LASER-INDUCED ACOUSTIC DESORPTION ATMOSPHERIC PRESSURE PHOTOIONIZATION FT-ICR MASS SPECTROMETRY 73 Introduction 73 4.1.1 Petroleum 73 4.1.2 Fourier-transform ion-cyclotron resonance (FT-ICR) mass spectrometry 76 4.1.3 Ion-source technology in petroleomics 78 Experimental 81 4.2.1 Samples and Materials 81 4.2.2 Sample preparation 82 4.2.3 LIAD-APPI Instrumentation 83 4.2.4 FT-ICR Mass Spectrometry Instrumentation 84 4.2.5 Data Analysis 85 Results and Discussion 87 4.3.1 Hydrocarbon Standards 87 4.3.2 NIST SRM 2779 – Macondo well oil 89 4.3.3 Khatyspyt formation shale extract 95 4.3.4 Azerbaijan Heavy Sweet (AH) and Venezuelan Heavy Sour (VH) Crude Oils 97 Conclusion 102 Acknowledgements 103 Supplemental 103 CHAPTER 5. CONCLUSIONS AND OUTLOOK 112 Review of Results 112 Future Directions 115 Final Remarks 118 APPENDIX A. Basic Operation for LIAD-APPI FT-ICR MS Experimentation 120 A.1 Requirements: 120 A.2 Set Up and Run Procedure 120 vii A.3 Shutdown Procedure: 125 A.4 Spectral Formula Assignment Workflow Using Bruker Compass © Software Suite 125 APPENDIX B. MULTIVARIATE ANALYSIS OF MASS SPECTROMETRY DATA FROM CHEMICALLY COMPLEX FOOD SAMPLES – A DATA PROCESSING INVESTIGATION 130 B.1 Introduction 130 B.2 Incorrect Data Processing Approaches 132 B.3 Suggested Data Processing 133 B.4 Testing 137 B.5 Continued Steps 141 B.6 Python source-code 142 APPENDIX C. MHCD SOFTWARE, DESIGN NOTES, AND MAINTENANCE 144 C.1 Software Requirements 144 C.2 Software Operation 144 C.3 Device Design, Geometry, and Fabrication 145 C.4 Maintenance - Electrode Replacement 146 C.5 Design Notes – Pressure Simulations 148 APPENDIX D. OPERATING PROCEDURE FOR THE IMATRIXSPRAY OPEN-SOURCE DEPOSITION SYSTEM 150 APPENDIX E. PYTHON CODE FOR PETROLEOMICS PLOT GENERATION 152 REFERENCES 154 viii LIST OF TABLES Table 1-1: Common excimer emissions from rare gases [74]. ......................................... 21 Table 2-1: Lowest Detected Amounts of Cholesterol and α-tocopherol. Foil coverage values were calculated using parameters from the iMatrixSpray system and relative error based on inhomogeneity of sample and uncertainty in laser spot size. ............................ 38 Table 3-1: Primary relative ion abundances for lipid standard compounds. .................... 60 Table 3-2: Table of assigned sterols to observed signals in extra-virgin olive oil. .......... 65 Table 4-1: Compound Identification Results for all Crude Oil Samples .......................... 98 Table 4-2: ESI Calibration Result ................................................................................... 107 Table 4-3: Physical Properties of Azerbaijan Aromatic-Naphthenic Crude Oil............. 108 Table 4-4: Bulk Chemical Properties of Azerbaijan Aromatic-Naphthenic Crude Oil .. 108 Table 4-5: Physical Properties of Venezuelan Heavy Sour Crude Oil (Reference ID: OGJ 99) ................................................................................................................................... 109 Table 4-6: Bulk Chemical and Trace Element Properties of Venezuelan Heavy Sour Crude Oil (Reference ID: OGJ 99) ................................................................................. 110 ix LIST OF FIGURES Figure 1-1: Common APPI dopants and their ionization potentials. .................................. 7 Figure 1-2: LIAD schematic illustrating molecular species (red-orange circles) being desorbed from an area opposite of the side of the laser strike. ......................................... 12 Figure 1-3: Visualization of a Townsend avalanche. Blue lines indicate path of incident electron where as red lines indicate path of the liberated electron. The yellow stars indicate the ionization event itself. ................................................................................... 16 Figure 1-4: Paschen curve for Neon gas. Where 풃 = 130 V cm-1 Torr-1, 풂 = 1.84 cm-1 Torr-1, and 휸 = 0.8 ............................................................................................................. 17 Figure 1-5: A generalized plot of current vs. voltage across multiple plasma regimes. Pink box indicates the glow discharge regime important to this work. ............................ 18 Figure 1-6: Schematic illustrating the Pendel Effect in a hollow cathode discharge device. The cathode is the only electrode shown for this figure. .................................................. 19 Figure 2-1: (a) Exploded view of the 3D model of the microplasma VUV device. (b) Circuit diagram with including ballast resistor (R1 = 97 kΩ) and voltage divider used for observing the discharge potential (R2 = 21 MΩ, R3 = 560 kΩ). (c) Picture of the device taken following fabrication. .............................................................................................. 27
Details
-
File Typepdf
-
Upload Time-
-
Content LanguagesEnglish
-
Upload UserAnonymous/Not logged-in
-
File Pages189 Page
-
File Size-