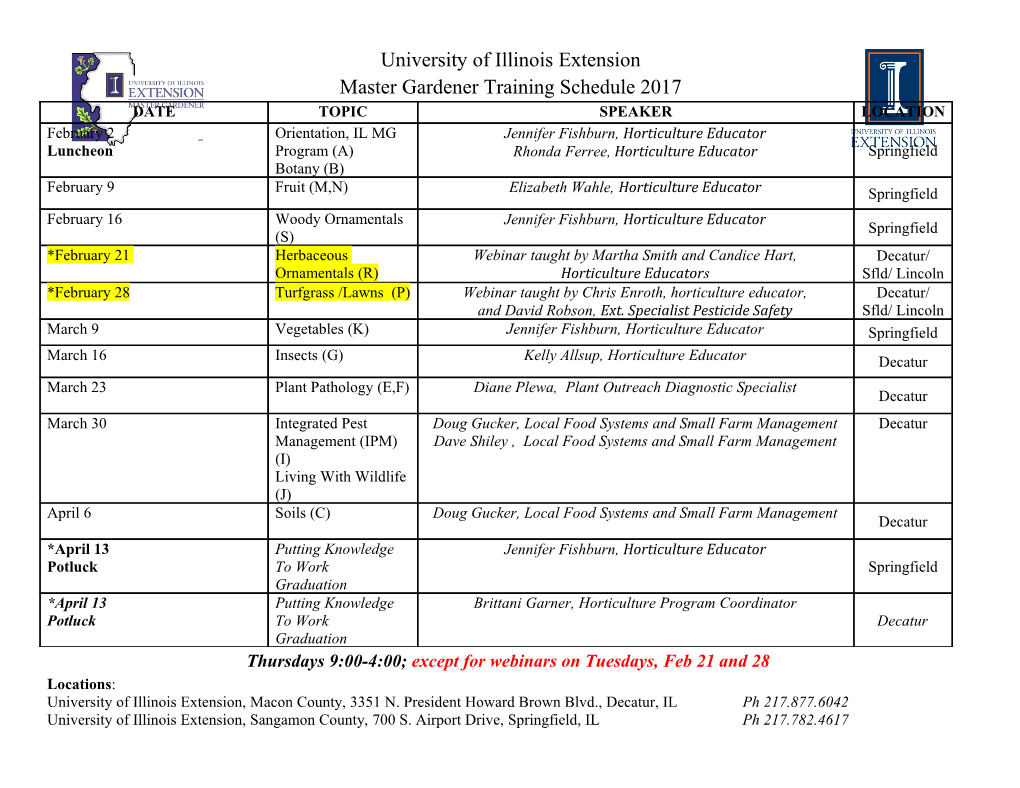
BMC Microbiology BioMed Central Research article Open Access Moraxella osloensis Gene Expression in the Slug Host Deroceras reticulatum Ruisheng An1, Srinand Sreevatsan2 and Parwinder S Grewal*1 Address: 1Entomology Department, The Ohio State University, Wooster, OH 44691, USA and 2Veterinary Population Medicine Department, University of Minnesota, St. Paul, MN 55108, USA Email: Ruisheng An - [email protected]; Srinand Sreevatsan - [email protected]; Parwinder S Grewal* - [email protected] * Corresponding author Published: 28 January 2008 Received: 8 March 2007 Accepted: 28 January 2008 BMC Microbiology 2008, 8:19 doi:10.1186/1471-2180-8-19 This article is available from: http://www.biomedcentral.com/1471-2180/8/19 © 2008 An et al; licensee BioMed Central Ltd. This is an Open Access article distributed under the terms of the Creative Commons Attribution License (http://creativecommons.org/licenses/by/2.0), which permits unrestricted use, distribution, and reproduction in any medium, provided the original work is properly cited. Abstract Background: The bacterium Moraxella osloensis is a mutualistic symbiont of the slug-parasitic nematode Phasmarhabditis hermaphrodita. In nature, P. hermaphrodita vectors M. osloensis into the shell cavity of the slug host Deroceras reticulatum in which the bacteria multiply and kill the slug. As M. osloensis is the main killing agent, genes expressed by M. osloensis in the slug are likely to play important roles in virulence. Studies on pathogenic interactions between bacteria and lower order hosts are few, but such studies have the potential to shed light on the evolution of bacterial virulence. Therefore, we investigated such an interaction by determining gene expression of M. osloensis in its slug host D. reticulatum by selectively capturing transcribed sequences. Results: Thirteen M. osloensis genes were identified to be up-regulated post infection in D. reticulatum. Compared to the in vitro expressed genes in the stationary phase, we found that genes of ubiquinone synthetase (ubiS) and acyl-coA synthetase (acs) were up-regulated in both D. reticulatum and stationary phase in vitro cultures, but the remaining 11 genes were exclusively expressed in D. reticulatum and are hence infection specific. Mutational analysis on genes of protein- disulfide isomerase (dsbC) and ubiS showed that the virulence of both mutants to slugs was markedly reduced and could be complemented. Further, compared to the growth rate of wild-type M. osloensis, the dsbC and ubiS mutants showed normal and reduced growth rate in vitro, respectively. Conclusion: We conclude that 11 out of the 13 up-regulated M. osloensis genes are infection specific. Distribution of these identified genes in various bacterial pathogens indicates that the virulence genes are conserved among different pathogen-host interactions. Mutagenesis, growth rate and virulence bioassays further confirmed that ubiS and dsbC genes play important roles in M. osloensis survival and virulence, respectively in D. reticulatum. Background contribute to our understanding of the evolution of bacte- As the dialog between a host and bacterium requires the rial virulence. There have been extensive studies on bacte- coordinated activity of many bacterial gene products in ria pathogenic to higher order hosts [2-7], particularly the response to the host [1], investigating the bacterial patho- zebrafish and mouse which have emerged as model ani- genesis in a diverse set of pathogen-host interactions can mals for the study of bacterial pathogenesis [8]. These Page 1 of 11 (page number not for citation purposes) BMC Microbiology 2008, 8:19 http://www.biomedcentral.com/1471-2180/8/19 studies have provided ample information on the virulence Several techniques have been developed to study bacterial genes essential for pathogenesis, yet we know little about genes that are expressed during infection or that are the origin and evolution of these genes. Characterizing the required for virulence in the host during infection. The bacterial genes expressed in hosts representing different most commonly used techniques include in vivo expres- evolutionary history may enable us to gain a better under- sion technology (IVET) [22], signature-tagged mutagene- standing of the evolution of bacterial virulence [1]. For sis (STM) [23] and differential fluorescence induction example, the pathogenic interaction between the nema- (DFI) [24]. Recently, the selective capture of transcribed tode Caenorhabditis elegans and bacterium Salmonella sequences (SCOTS) technique has been developed to enterica has showed a remarkable overlap between Salmo- study bacterial gene expression specifically in macrophage nella virulence factors required for human and nematode [25]. This technique has been used to identify bacterial pathogenesis [9]. While there are several reports of bacte- virulence factors, and has been demonstrated to be capa- rial illness in snakes, tortoises, and reptiles [10-13], little ble of identifying genes expressed in specific tissues of is known about bacteria-involved infections in a slug host infected animals [5,26]. More recently, microarray analy- [14]. sis has also been applied in monitoring bacterial gene expression during host infection [27]. As the host and bac- As lower order invertebrates, mollusks are proven to be terial cDNAs can be easily differentiated using SCOTS, excellent model systems for studies in neurophysiology, and this technique does not require prior genetic informa- behavioral ecology and population genetics [15]. The slug tion of the pathogen, we applied SCOTS to determine M. Deroceras reticulatum is one of the important mollusk osloensis gene expression in the slug host D. reticulatum at invertebrates. Moraxella osloensis is a gram-negative, oxi- two time points following infection. Because important dase positive, aerobic bacterium within the family Morax- changes in gene expression can occur in bacteria during ellaceae in the gamma subdivision of the purple bacteria. transition from active growth to stationary phase [5,28], This bacterium has recently been identified as one of the we also investigated differential gene expression of 72 h natural symbionts of a bacteria-feeding nematode, Phas- M. osloensis in the in vitro cultures (stationary phase) rela- marhabditis hermaphrodita (Rhabditida: Rhabditidae), tive to 24 h cultures (log phase) to confirm the infection which is a lethal endoparasite of slugs [16,17], including specificity of the in vivo expressed genes. Mutational anal- the slug D. reticulatum [18]. In nature, bacteria colonize yses (virulence of mutants to the slug and in vitro growth the gut of nematode infective juveniles (IJs) which repre- rate of mutants) were also carried out to examine the roles sent a specialized stage of development adapted for sur- of selected genes. vival in the unfavorable environment. The IJs seek out and enter the slug's shell cavity through the posterior mantle Results region. Once inside the shell cavity, the bacteria are Differentially in vivo expressed genes released, and the IJs resume growth, feeding on the multi- To identify M. osloensis transcripts specifically expressed in plying bacteria [18-20]. The infected slugs die in 4–10 D. reticulatum, we injected slugs with 5 × 107 bacterial days, and the nematodes colonize the entire cadaver and cells. We evaluated colonization by analyzing M. osloensis produce next generation IJs which leave the cadaver to cell counts, and found that bacterial counts varied from seek a new host [18]. 105 to 108 colony-forming units (CFU) per D. reticulatum 48 h or 96 h post-inoculation, indicating colonization The lethality of these nematodes to slugs has been shown and persistence of the injected bacteria in the slug. Tran- to correlate with the number of M. osloensis cells carried by scripts expressed by M. osloensis in the slug at 48 h and 96 IJs [20]. Tan and Grewal [20] demonstrated that the 72 h h post inoculation were identified by subjecting the in vivo old M. osloensis cultures inoculated into the shell cavity cDNAs to three iterations of SCOTS in the presence of the were highly pathogenic to the slug. They further reported transcripts expressed by 48 h late log-phase M. osloensis in that M. osloensis produced an endotoxin which was iden- vitro cultured in the Brain Heart Infusion (BHI) (Difco) tified to be a rough type lipopolysaccharide (LPS) with a medium. The enriched cDNAs were cloned into a TA vec- molecular weight of 5300 KD, and the purified M. osloen- tor. Each individual clone was further screened by dot blot sis LPS was toxic to the slug with an estimated 50% lethal hybridization (Fig. 1). The individual clones with signals dose of 48 µg when injected into the shell cavity [21]. stronger than or present on the blot hybridized to the in Although these studies laid the foundation for the bacte- vivo cDNAs compared to that with signals on the blot ria-slug interaction, the virulence mechanisms of M. hybridized to in vitro cDNAs were chosen for nucleotide osloensis that result in pathogenesis and slug mortality are sequencing and analysis. A total of 97 clones (27 from 48 not established. The present study was designed to deter- h post inoculation, and 70 from 96 h post inoculation) mine the molecular and genetic basis of M. osloensis viru- were sequenced. These screened cDNAs represented the lence to the slug D. reticulatum. differentially expressed genes within the slug but in lower abundance or absent in the 48 h in vitro cultures. A frac- Page 2 of 11 (page number not for citation purposes) BMC Microbiology 2008, 8:19 http://www.biomedcentral.com/1471-2180/8/19 bases, and is possibly novel. M1, M2 and M4 genes con- tain translated sequences of "Gly-Asp-Pro-Asp" repeats, and are similar to membrane proteins. Sequence M5 shows similarity with the gene encoding protein-disulfide isomerase (DsbC) in other bacteria. Sequences M6, M8, and M10 have similarity to genes that encode iron regula- tion related proteins in other bacteria; thus, the identifica- tion of these genes suggests that iron availability in slug host may be limited.
Details
-
File Typepdf
-
Upload Time-
-
Content LanguagesEnglish
-
Upload UserAnonymous/Not logged-in
-
File Pages11 Page
-
File Size-