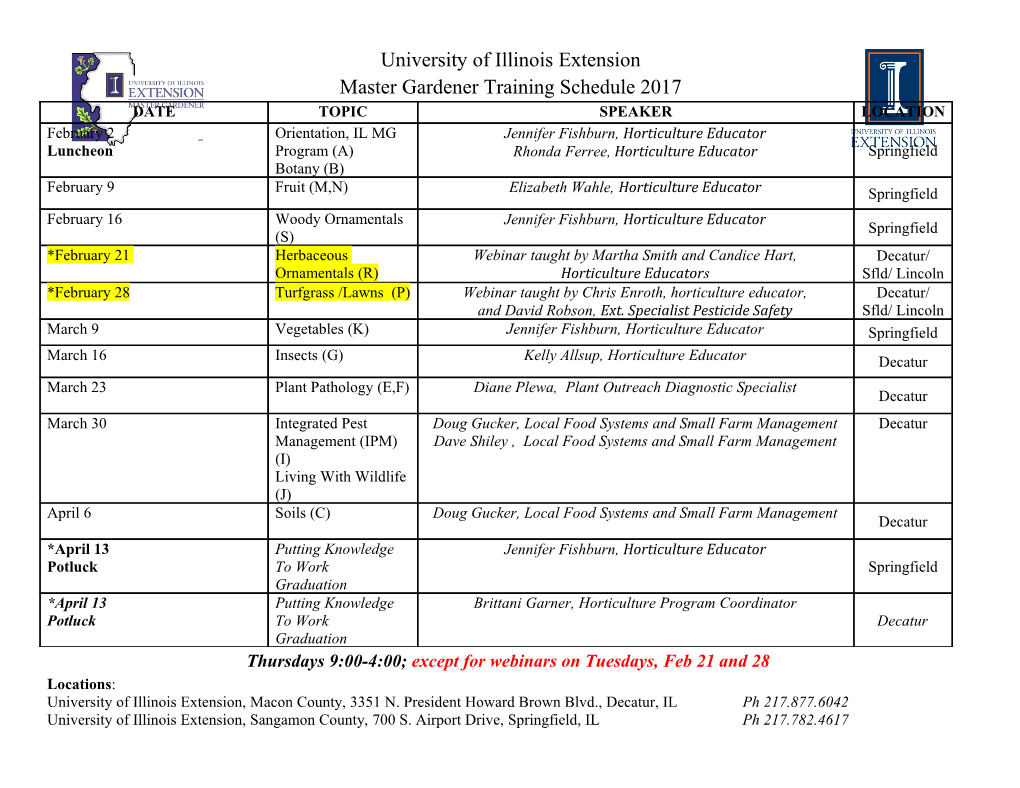
Hindawi Journal of Sensors Volume 2019, Article ID 6074046, 8 pages https://doi.org/10.1155/2019/6074046 Research Article Facile Preparation of g-C3N4-WO3 Composite Gas Sensing Materials with Enhanced Gas Sensing Selectivity to Acetone Xiangfeng Chu ,1 Junsong Liu,1 Shiming Liang ,2 Linshan Bai,1 Yongping Dong,1 and Mauro Epifani 3 1School of Chemistry and Chemical Engineering, Anhui University of Technology, Maanshan 243002, China 2School of Materials Science and Engineering, Linyi University, Linyi 276005, China 3Consiglio Nazionale delle Ricerche, Istituto per la Microelettronica ed i Microsistemi, Via Monteroni I-73100 Lecce, Italy Correspondence should be addressed to Xiangfeng Chu; [email protected] and Shiming Liang; [email protected] Received 23 May 2019; Accepted 30 August 2019; Published 4 December 2019 Academic Editor: Bruno C. Janegitz Copyright © 2019 Xiangfeng Chu et al. This is an open access article distributed under the Creative Commons Attribution License, which permits unrestricted use, distribution, and reproduction in any medium, provided the original work is properly cited. In this paper, g-C3N4-WO3 composite materials were prepared by hydrothermal processing. The composites were characterized by means of X-ray powder diffraction (XRD), scanning electron microscopy (SEM), Fourier transform infrared spectroscopy (FTIR), X-ray photoelectron spectroscopy (XPS), and N2 adsorption-desorption, respectively. The gas sensing properties of the composites were investigated. The results indicated that the addition of appropriate amount of g-C3N4 to WO3 could improve the response and selectivity to acetone. The sensor based on 2 wt% g-C3N4-WO3 composite showed the best gas sensing performances. When operating at optimum temperature of 310°C, the responses to 1000 ppm and 0.5 ppm acetone were 58.2 and 1.6, respectively, S S and the ratio of the 1000 ppm acetone to 1000 ppm ethanol reached 3.7. 1. Introduction particles which had a high response (S = 150–280) even to – ° dilute NO2 (50 1000 ppb) in air at 200 C. A study by Ma Graphitic carbon nitride (g-C3N4) nanomaterial exhibits a et al. [5] showed that WO3 nanoplates obtained through a п stable layered structure and -conjugated s-triazine unit topochemical transformation of the corresponding H2WO4 composed of sp2 hybridized carbon atoms and sp2 hybridized precursor exhibited high response to ethanol while operating ° nitrogen atom. g-C3N4 nanosheets have attracted the atten- at 300 C. tion of researchers in recent years for its peculiar properties The photocatalytic activity of g-C3N4-WO3 nanocom- as a semiconductor such as immense specific surface area posites also has been reported by many researchers [6–9]; [1]. Wang et al. [2] prepared g-C3N4 by thermal treatment the photocatalytic performances of WO3/g-C3N4 nanocom- of glucose and urea, and the p-type sensor based on g-C3N4 posites were higher than those of pure WO3 and pure ff exhibited good response to NO2 at room temperature. g-C3N4, which were attributed to the synergistic e ect of As a gas sensing material, WO3 has been paid much WO3 and pure g-C3N4. A study by Zhang et al. [10] showed α attention in the past decade. Cho et al. [3] used ultrasonic that the ethanol sensing performance of -Fe2O3/g-C3N4 α spray pyrolysis to prepare WO3 hollow spheres using a citric nanocomposites was better than that of pure -Fe2O3 and α acid-containing precursor solution; the WO3 hollow spheres g-C3N4, which could be caused by porous -Fe2O3 nanotubes exhibited a high response and good gas sensing selectivity wrapped by lamellar g-C3N4 nanostructures resulting in the to trimethylamine, but the sensor exhibited a depressed formation of heterojunction. Cao et al. [11] reported that fi response to NO2. Kida et al. [4] used acidi cation of Na2WO4 the gas sensing response and selectivity to ethanol could also fi with H2SO4 solution to prepare lamellar-structured WO3 be enhanced by modi cation of g-C3N4 nanosheets. The 2 Journal of Sensors combination of WO3 and g-C3N4 may exhibit good gas sens- 3. Results and Discussion ing properties, which to the best of our knowledge has not been reported to date. 3.1. Characterization. Figure 1 shows the XRD patterns of In this paper, we report the preparation of g-C N -WO pure g-C3N4, g-C3N4-WO3 (S-1, S-2, S-3, and S-4), and 3 4 3 fi ff WO3. Two signi cant di raction peaks were observed at nanocomposites through a hydrothermal method and the ° ° investigation of their gas sensing properties. Analysis showed 13.23 and 27.86 in the XRD pattern of g-C3N4, indicating the (100) and (002) planes of layered g-C3N4; the weaker that 2 wt% g-C3N4-WO3 nanocomposite responded highly ° and selectively to acetone. peak at 13.23 indicates the in-planar tris-s-triazine structural packing, and the stronger peak at 27.86° corresponds to the – 2. Materials and Methods stacked of the aromatic systems between layers [6 8, 14]. All the diffraction peaks observed in XRD patterns of S-0, S-1, S-2, S-3, and S-4 could be indexed to the standard data 2.1. Material Preparation and Characterization. g-C3N4 was ° ff prepared by heating 2.0 g melamine in an oven at 520 C for of WO3 (JCPDS 43-1035). The di raction peaks of g-C3N4 5 hours, while keeping the heating rate at 5°C/min, which could not be found because of the low concentration of was similar to that reported in the literature [12]. After cool- g-C3N4 in the composites [14]. It was reported that the dif- ing to room temperature naturally, the product was purified fraction peaks of g-C3N4 did not appear in the XRD patterns ° with ethanol, following which it was dried at 60 C for 24 h of g-C3N4-WO3 composites when the content of WO3 was succeeded by milling. higher than 10 wt% [15]. The average crystallite sizes were For preparing the nanocomposites, a certain amount of calculated by the Scherrer formula: as-prepared g-C3N4 was added to 40 mL deionized water and sonicated for 1 hour to obtain a g-C N suspension. Kλ 3 4 D = , ð1Þ 0.0025 mol Na2WO4·2H2O was dissolved in 20 mL deionized β cos θ water, and 4 mL concentrated hydrochloric acid was added dropwise in the Na2WO4 solution slowly while stirring where D is the crystallite size, K is the instrument correction resulting in the formation of H2WO4; the g-C3N4 suspension factor, γ is the Cu-Kα wavelength (0.151418 nm), β is the was added slowly to H2WO4 while stirring. The mixture was θ fl full width at half maximum of the peaks, and is the posi- sealed in a 100 mL Te on-lined stainless steel autoclave and tion of the peaks. The average crystallite sizes of WO ° fi 3 in heated at 200 C for 24 h; the obtained precipitate was ltered S-0, S-1, S-2, S-3, and S-4 were 54.9, 62.6, 44.4, 44.3, and and washed with distilled water and ethanol, followed by 50.2 nm, respectively. The calculated particle size of g-C ° fi 3N4 drying in air at 80 C for 24 hours; nally, the g-C3N4-WO3 was about 5.7 nm. composite was obtained. The weight ratios of g-C3N4 pow- Figure 2 exhibits the SEM images of g-C3N4, pure WO3 ders/WO3 (the weight of WO3 was calculated according to (S-0), and g-C3N4-WO3 (S-2). The morphology of g-C3N4 the weight of Na2WO4·2H2O) were 0 wt%, 1 wt%, 2 wt%, was a sheet that consisted of small particles, and the particle 3 wt%, and 4 wt% (the samples were labeled as S-0, S-1, S-2, sizes were about 200 nm. The WO particle sizes in WO and S-3, and S-4, respectively). 3 3 ff α g-C3N4-WO3 were between 100 and 400 nm; the addition of X-ray di raction (XRD, Bruker D8 Advance, Cu-K g-C N in the composite had no obvious influence on the radiation: λ =0:15418 nm), operating at 40 kV and 30 mA 3 4 θ ° ° particle size of WO3. The particle sizes obtained from SEM in a 2 range from 10 to 70 at room temperature, was used images were larger than those calculated from the Scherrer to analyze the crystal structure of g-C3N4-WO3 nanocom- formula, which manifested that the particles observed by posites. A scanning electron microscope (SEM, Hitachi S- SEM in g-C3N4, pure WO3, and g-C3N4-WO3 were aggre- 4800 microscope), with an accelerating voltage of 10 kV, gates of smaller particles. was used to characterize the surface morphology of the sam- The FTIR spectra of WO3 and g-C3N4-WO3 (S-2) are ples. Fourier transform infrared spectroscopy (FTIR, Nicolet shown in Figure 3. The absorption peaks at 763, 822, and 6700 FTIR Spectrometer) spectra were recorded by the KBr 935 cm-1 in the spectra of two samples originated from pellet technique in the range 400–4000 cm-1. The chemical stretching vibrations of O-W-O in WO3 [9, 15, 16]; the peaks species of elements were analyzed by X-ray photoelectron -1 at 1632 and 3436 cm in the FTIR spectra of WO3 and spectroscopy (XPS, Thermo ESCALAB250Xi) with all of g-C N -WO (S-2) resulted from the vibration of bended the binding energies corrected according to contaminant car- 3 4 3 : fi H-O-H and stretched O-H of absorbed H2O on the material bon (C1s = 284 6eV).
Details
-
File Typepdf
-
Upload Time-
-
Content LanguagesEnglish
-
Upload UserAnonymous/Not logged-in
-
File Pages9 Page
-
File Size-