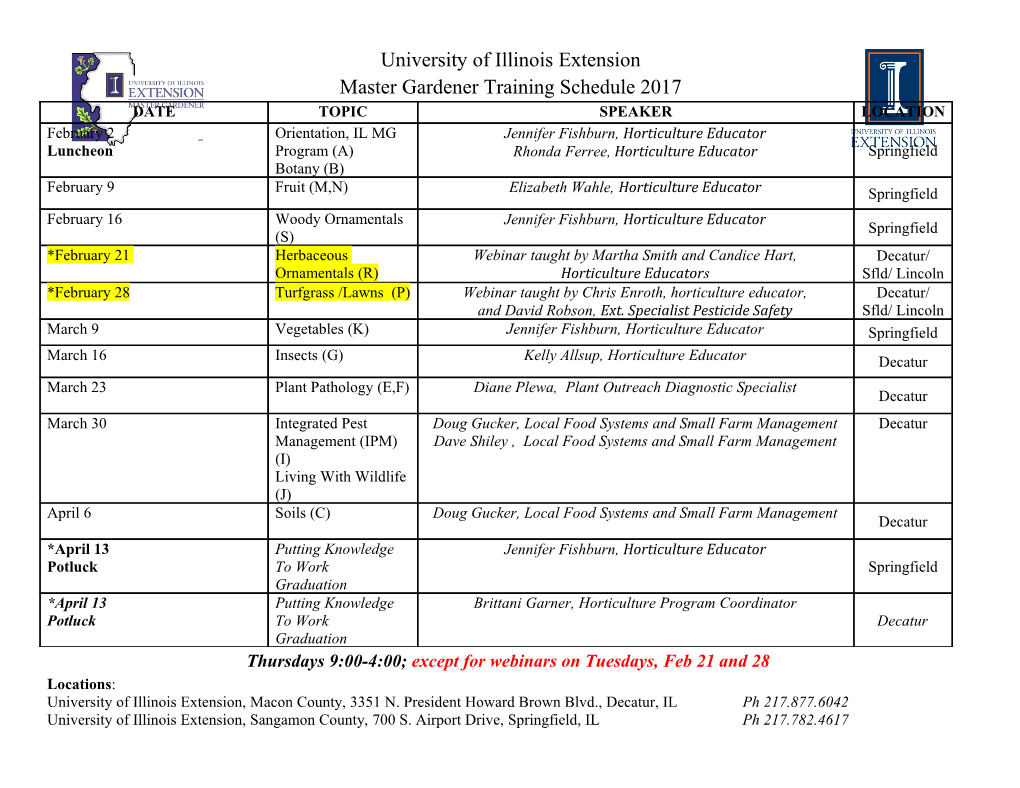
Migratory Reductive Cross-Coupling via Dual Nickel Metathesis Yuqiang Li, Binzhi Zhao, Long Peng, Guoyin Yin* The Institute for Advanced Studies, Wuhan University, Wuhan, Hubei 430072, P. R. China Abstract: Cross-electrophile coupling has been developed into a practical approach for the construction of carbon- carbon bonds, wherein nickel catalysis has been widely employed. Mechanistically, a catalytic cycle involving sequentially selective oxidative addition or radical chain process is proposed. Although the catalytic cycle of dual nickel metathesis has been discussed in several important works, none thinks this pathway is possible. In this manuscript, we present a thorough mechanistic study by a series of designed experiments toward the nickel-catalyzed migratory reductive cross-coupling. The results suggest that a catalytic cycle involving two organonickel(II) species metathesis as a key step, operates in this reaction. Moreover, we provide a discussion on the difference between the nickel-catalyzed migratory reductive cross-couplings and the classical ones. Additionally, based on the mechanistic finding, a new catalytic system has also been developed, which allows the use of electron-deficient aryl halides as starting materials, affording the migratory cross-coupling products efficiently. Keywords: nickel catalysis, dual nickel metathesis, mechanism Transition metal-catalyzed cross-coupling reactions have extraordinary performance in cross-electrophile coupling revolutionized chemical bond formation strategies.1 Among reactions and significant progress has been achieved by Weix,4 these, the cross-coupling of two electrophiles, avoiding Gong,5 , Reisman6 and others. Mechanistically, a catalytic cycle handling air- and/or moisture-sensitive metal reagents, has been involving sequential selective oxidative addition was proposed developed into well-recognized, practical protocols in organic for most of those reactions (Figure 1A).7 Weix and coworkers synthesis in the past decade.2 Nickel catalysis3 exhibits have initially demonstrated efficient reductive alkyl-aryl 1 coupling reactions, and the selective oxidative addition of aryl migratory cross-coupling reactions. The experimental results halides followed by a radical chain process has been firmly support a catalytic cycle with dual nickel(II) metathesis as a key established to rationalize this transformation (Figure 1B).8-9 step (Figure 1C).20 Subsequently, radical chain catalytic cycles have been proposed, particularly in those reactions involving alkyl electrophiles.10-11 Both catalytic cycles are characterized by selective oxidative addition. These two catalytic cycles have prevailed in Ni- catalyzed reductive cross-electrophile coupling reactions, and laid a solid foundation for reaction design in this area.12 Recently, exploring transformations involving metal migration stimulated great interest within the synthetic community.13 In this context, Zhu14-15 and our group16-17 independently developed nickel-catalyzed reductive migratory cross-coupling of alkyl halides with aryl halides. A mechanism involving selective, sequential oxidative addition with Ni(I) chain- walking was proposed by Zhu and coworkers,15 whilst a catalytic cycle involving a radical chain process and Ni(III) chain-walking was proposed to rationalize our previous results.17 However, the mechanism of these type transformations has still not been fully elucidated.18 First, reductive elimination is more favorable than -hydride Figure 1. Mechanism of Ni-Catalyzed Migratory Cross-Coupling elimination at the high-valent Ni(III) species. Second, it is of Alkyl Electrophiles difficult to explain the formation of migratory Suzuki-Miyaura Results coupling products via the Ni(I) chain-walking pathway.19 Third, the fact that a change of ligand results in a change of Based on previous mechanistic studies of nickel catalysis,21 regioselectivity in our reactions still lacks a reasonable several possible catalytic cycles were proposed for the explanation. migratory reductive cross coupling (Figure 2): A) sequential oxidative addition with Ni(I) chain-walking;14, 22 B) sequential To address the questions mentioned above, we continued our oxidative addition with Ni(II) chain-walking;19, 23 C) radical mechanistic investigation into the nickel-catalyzed reductive 2 chain catalytic cycle with Ni(III) chain-walking;16 D) promote the homocoupling of 2a in an excellent yield (93%), transmetallation between Ni(I)/Ni(III) with Ni(I) chain-walking; whilst the reaction with L1 was slow and afforded the same E) transmetallation between Ni(I)/Ni(II) with Ni(II) chain- product in only 33% yield after 18 hours (Figure 3F and 3G).27 walking; F) transmetallation between Ni(II)/Ni(II) with Ni(II) These results are consistent with an early report from chain-walking.19, 24 Yamamoto and coworkers, which demonstrated that the dual Ar-Ni(II) complex metathesis was the rate-determining step in Products Analysis. We chose the cross-coupling of 1a with 2a the homocoupling reaction under similar conditions.24 Recently, (the ratio is 1:1) as model reaction. As shown in Figure 3A, the Martin group also showed that the complex of Ar-Ni(II)L1- besides cross-coupling products 3a and 4a, a small amount of Br was very stable in coordinating polar solvents.28 migratory homocoupling product 5a and homocoupling product 6a, as well as 19% of olefin 7a were also observed under our Considering that there is only one substrate involved in the prior conditions.16 homo-coupling reaction of electrophilic reagent due to the heterogeneous system, we can assess the order in electrophile Comparison of Ligands. In order to gain more insight on from single reaction profiles. We found that the initial rate of ligand effect on oxidative addition of nickel catalyst to the reactions with BC as ligand had a first-order dependence on electrophile, nickel-catalyzed homocoupling of each the electrophile, but zeroth order-dependence was observed in electrophile in the presence of distinct ligands was subsequently the reactions with L1 as ligand (Supplementary Fig.1-4). These studied. As shown in Figure 3B, the product 5a was isolated in results suggest that the oxidative addition is the rate- 58% yield with bathocuproine (BC) as ligand using 1a as determining step (RDS) in the homocoupling reactions with BC substrate, which suggests the formation of benzyl nickel species. as ligand, but not in the ones with L1. This finding reveals that, Homocoupling of 1a was also investigated using 5,5’- likely owing to the steric hindrance of ortho-methyl groups, the dimethylbipyridine (L1), which afforded the original-site oxidative addition is more difficult in the reactions with BC than 25 coupling product 5b in 46% yield (Figure 3C). Notably, these with L1. two reactions exhibit similar rates (Figure 3D). Meanwhile, phenyl bromide (2a) was also investigated with these two ligands.24, 26 As presented in Figure 3E, BC could efficiently 3 Figure 2. Possible Catalytic Cycles for the Migratory Reductive Cross-Coupling . Competitive Oxidative Addition. In a radical chain pathway, couplings.9 However, when the aryl coupling partner was reaction selectivity derives from the difference between the switched to 4-methoxylphenyl bromide (2c), the consumption rates of oxidative addition of two electrophiles and metathesis of aryl electrophile is slower than the alkyl electrophile (Figure of the readily generated Ar-Ni(II) species.9 In order to get more 3H). These results indicate that the relative reactivity is affected information on the oxidative addition of this migratory reaction, by the electronic property of the aryl electrophile, which reveals oxidative addition experiments were conducted. We found that competitive oxidative addition takes place in the reaction. It is in the reaction of 3-phenylpropyl bromide (1a) with 4- worth noting that compared with the results of classical phenoxylpheneyl bromide (2b) (Figure 3H), the consumption of reductive coupling reactions, the rate of consumption of two aryl bromide was faster than that of alkyl bromide. This result electrophiles under these conditions does not show a large is consistent with the observations in classical cross-electrophile difference. 4 Figure 3. A) Modified Reaction Conditions. B) Homocoupling of Alkyl Bromide Catalyzed by NiI2/BC. C) Homocoupling of Alkyl Bromide Catalyzed by NiI2/L1. D) Time Curve of Reaction B and C. E) Homocoupling of Aryl Bromide Catalyzed by NiI2/BC. F) Homocoupling of Aryl Bromide Catalyzed by NiI2/L1. G) Time Curve of Reaction E and F. H) Stoichiometric Reactions. I) Reactions Conducted with Different Ratio of 1a/2a. J) Reactions Conducted with Different Ratio of 1b/2c. 5 cross-coupling product 3d appeared about 30 min later, when We surmised that if competitive oxidative addition takes place most of 2d was consumed. To our surprise, 5a, the in the reaction, the reaction rate should be not only altered along homocoupling product of 1a, was not detected until most of 2d with the concentrations of substrates, but also along with the was consumed. substrates themselves. To verify this idea, a series of kinetic experiments were carried out. However, the consumption of alkyl electrophile was faster than aryl electrophile, when 1b reacted with an electron-rich aryl Kinetic Analysis. As illustrated in Figure 3I, for the cross bromide (2c, Supplementary Fig. 11). Accordingly, the coupling of 1a with 2a, we found that the reaction rate increased homocoupling product of the alkyl electrophile (5a) was with
Details
-
File Typepdf
-
Upload Time-
-
Content LanguagesEnglish
-
Upload UserAnonymous/Not logged-in
-
File Pages20 Page
-
File Size-