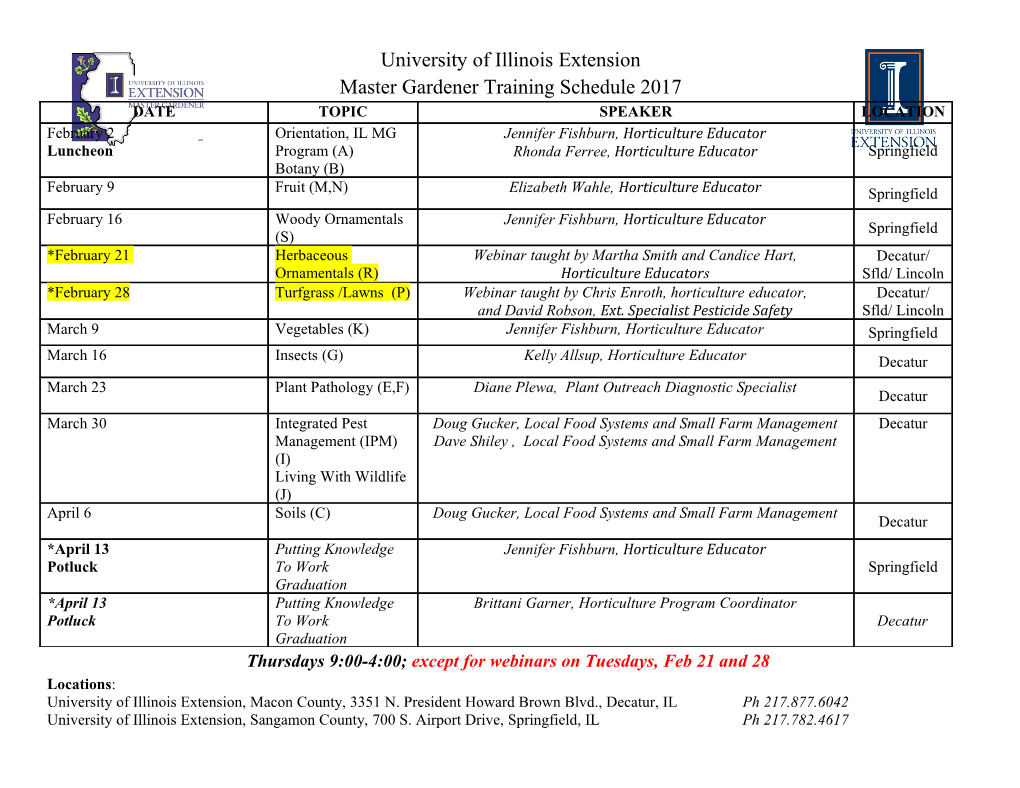
Geophys. J. Int. (2009) doi: 10.1111/j.1365-246X.2009.04178.x Upper-mantle low-velocity zone structure beneath the Kaapvaal craton from S-wave receiver functions Samantha E. Hansen,1 Andrew A. Nyblade,2 Jordi Julia,` 1 Paul H.G.M. Dirks3 and Raymond J. Durrheim3 1Department of Geosciences, 407 Deike Bldg., Pennsylvania State University, University Park, PA 16802, USA. E-mail: [email protected] 2Department of Geosciences, 447 Deike Bldg., Pennsylvania State University, University Park, PA 16802, USA 3School of Geosciences, University of the Witwatersrand, Private Bag X3, Wits 2050, South Africa Accepted 2009 March 10. Received 2009 February 9; in original form 2008 October 3 SUMMARY The southern African Plateau is marked by anomalously high elevations, reaching 1–2 km above sea level, and there is much debate as to whether this topography is compensated by a lower mantle source or by elevated temperatures in the upper mantle. In this study, we use S-wave receiver functions (SRFs) to estimate the lithospheric thickness and sublithospheric mantle velocity structure beneath the Kaapvaal craton, which forms the core of the Plateau. To fit the SRF data, a low-velocity zone (LVZ) is required below a ∼160-km-thick lithospheric lid, but the LVZ is no thicker than ∼90 km. Although the lid thickness obtained is thinner than that reported in previous SRF studies, neither the lid thickness nor the shear velocity decrease (∼4.5%) associated with the LVZ is anomalous compared to other cratonic environ- ments. Therefore, we conclude that elevated temperatures in the sublithospheric upper mantle contribute little support to the high elevations in this region of southern Africa. Key words: Seismicity and tectonics; Cratons; Africa. lid with lower than average lid velocities or as an anomalously INTRODUCTION large or an anomalously slow low-velocity zone (LVZ). Results The depth extent of continental lithosphere beneath Archean and from modelling regional P waves, as well as body wave tomogra- Proterozoic shields has been debated for many decades (e.g., phy, show high velocities extending to 300–400 km depth beneath MacDonald 1963; Jordan 1975), and much of this debate has re- southern Africa, with little evidence for a LVZ beneath the region volved around the upper-mantle structure beneath the Kaapvaal (Zhao et al. 1999; James et al. 2001; Fouch et al. 2004). Inversion GJI Tectonics and geodynamics craton in southern Africa (Fig. 1). Unlike many shields, which of fundamental mode surface-wave phase delays displays radial display average elevations 400–500 m above sea level, the sur- anisotropy throughout the upper mantle but no LVZ, indicating a face topography in southern Africa reaches 1–2 km above sea thick lithosphere (Freybourger et al. 2001; Saltzer 2002). Inversion level, with residual bathymetry in the surrounding oceans of more of both two-station fundamental-mode Rayleigh wave phase veloc- than 500 m (Nyblade & Robinson 1994). There is much contro- ities and multiscale finite-frequency Rayleigh wave phase residuals versy as to how this area of high topography, termed the ‘African yield similar results (Larson et al. 2006; Chevrot & Zhao 2007). Superswell’ (Nyblade & Robinson 1994), is compensated and However, other studies using multimode high-frequency surface whether it originates from either a lower- or upper-mantle source wave data (Priestley 1999; Priestley et al. 2006) and Rayleigh wave of buoyancy. Lithgow-Bertelloni & Silver (1998) and Gurnis et al. tomography (Li & Burke 2006) find a high velocity lid beneath (2000), for example, suggested that dynamic topography generated much of southern Africa, with a lid thickness between 160–200 by a large-scale upwelling originating at the core–mantle boundary km for the Kaapvaal craton, underlain by a ∼150 km thick LVZ supports the high elevations across southern Africa. Other studies associated with a ∼4% shear velocity (VS) decrease. Joint mod- have suggested that buoyancy from a thermal anomaly in either the elling of regional SH waves and mineral physics data also suggests lithosphere (Nyblade & Sleep 2003) or the asthenosphere (Burke a pronounced LVZ beneath southern Africa, with VS reductions of et al. 2003; Li & Burke 2006; Burke & Gunnell 2008) might support at least 5% beneath a 150-km-thick, high-velocity lithospheric lid the superswell. (Wang et al. 2008). A 150–200-km-thick lid beneath the Kaapvaal To distinguish between these competing models, many studies craton is consistent with lithospheric thickness estimates inferred have examined the upper-mantle velocity structure beneath parts of from heat flow measurements and from pressure–temperature esti- southern Africa. Evidence of a thermal anomaly in the uppermost mates, based on kimberlite nodule data (Ballard & Pollack 1988; mantle may be manifested either as an anomalously thin lithospheric Jones 1988; Rudnick & Nyblade 1999; Artemieva & Mooney 2001; C 2009 The Authors 1 Journal compilation C 2009 RAS 2 S. E. Hansen et al. (http://africaarray.psu.edu) and Global Seismographic Network (GSN) broad-band stations throughout the Kaapvaal craton were used in this study (Fig. 1). The SASE stations were deployed between 1997 April and 1999 July, and most of the AfricaArray stations were installed in early- to mid-2006; so, each of these net- works provides about 1.5–2 yr of data. Significantly more data were recorded by GSN station BOSA, which has been operating since 1993 February. We selected S waves with high signal-to-noise ra- tios recorded at these stations from earthquakes with magnitudes larger than 5.7, depths less than 240 km and distances between 60◦ and 80◦. It has been shown that this depth and distance criteria iso- lates true Sp phases from potential contamination by other P-wave phases (Wilson et al. 2006). Our event selection differs from that used in the Kaapvaal SRF study by Wittlinger & Farra (2007), who incorporated events with epicentral distances up to 110◦. Waveforms were first rotated from the N–E–Z to the R–T–Z co- ordinate system using the event’s backazimuth and were visually inspected to pick the S-wave onset. The three-component records were then cut to focus on the section of the waveform that is 100 s prior to and 12 s after the S arrival. To detect Sp conversions, the data must be rotated around the incidence angle into the SH–SV–P coordinate system (Li et al. 2004). This second rotation is critical because if an incorrect incidence angle is used, noise can be signif- icantly enhanced, and converted phases may become undetectable. The optimal incidence angle was determined using the approach Figure 1. Stations from the SASE (black triangles) and AfricaArray (grey of Sodoudi (2005), as described by Hansen et al. (2007). Using triangles) networks, along with GSN station BOSA (white triangle), used in Ligorria & Ammon’s (1999) iterative time domain method, SRFs this study. Grey circles show conversion points at 160 km depth from events meeting the SRF criteria. Bold lines outline the boundaries of labelled are generated by deconvolving the SV component from the cor- tectonic terranes. responding P component. To make the SRFs directly comparable with PRFs, both the time axes and the amplitudes of the SRFs were Deen et al. 2006; Priestley et al. 2006), but these data sets do not reversed (e.g. Farra & Vinnik 2000; Li et al. 2004; Kumar et al. provide constraints on the nature of the LVZ. 2007). The frequency content of the receiver function is controlled In this study, we use the S-wave receiver function (SRF) tech- by the Gaussian width factor, a (Ligorria & Ammon 1999). Several nique (e.g., Farra & Vinnik 2000; Li et al. 2004; Kumar et al. 2007) values of a were examined; however, the best and most consistent to determine the depth to the lithosphere–asthenosphere boundary results were obtained using an a of 1.0. (LAB) by identifying S-to-P (Sp) conversions from discontinuities Once receiver functions were generated for all events at each sta- beneath seismic stations in the Kaapvaal craton. Unlike P-wave tion, the data set was subjected to a number of quality control crite- receiver functions (PRFs), where crustal multiples can mask con- ria not employed in previous SRF studies (e.g. Kumar et al. 2007; versions from the LAB, boundary conversions on SRFs can be more Wittlinger & Farra 2007). Each receiver function was first compared clearly identified because they arrive earlier than the direct S phase with previously determined PRFs at the same station to identify the whereas all crustal multiples arrive later (e.g. Farra & Vinnik 2000; crust–mantle boundary (Moho) conversion. Across our study area, Li et al. 2004; Kumar et al. 2007). SRFs have been used in several the crustal thickness is fairly uniform, ranging from about 36 to previous studies to investigate the lithospheric structure beneath 41 km (Nguuri et al. 2001; Kgaswane et al. 2006, 2008). Only southern Africa (Kumar et al. 2007; Wittlinger & Farra 2007), but SRFs that display a clear Moho conversion at the appropriate time new methodological approaches and data selection criteria warrant were used for further analysis. Next, the amplitude of the Moho a re-examination of those results. Our SRF analysis focuses on Sp conversion was examined. Forward modelling was used to predict conversions within the southern and central Kaapvaal craton to re- the expected Moho amplitude using published averaged velocities assess both the lithospheric thickness and upper-mantle velocity for the crust and upper mantle (Niu & James 2002; Larson et al. structure beneath this region. This approach provides new insight 2006; Li & Burke 2006; Nair et al. 2006; Wang et al. 2008). If the into what role the LVZ, if one exists, plays in supporting the high amplitude of the Moho conversion on the SRF was significantly too elevation in this region of the African Superswell.
Details
-
File Typepdf
-
Upload Time-
-
Content LanguagesEnglish
-
Upload UserAnonymous/Not logged-in
-
File Pages7 Page
-
File Size-