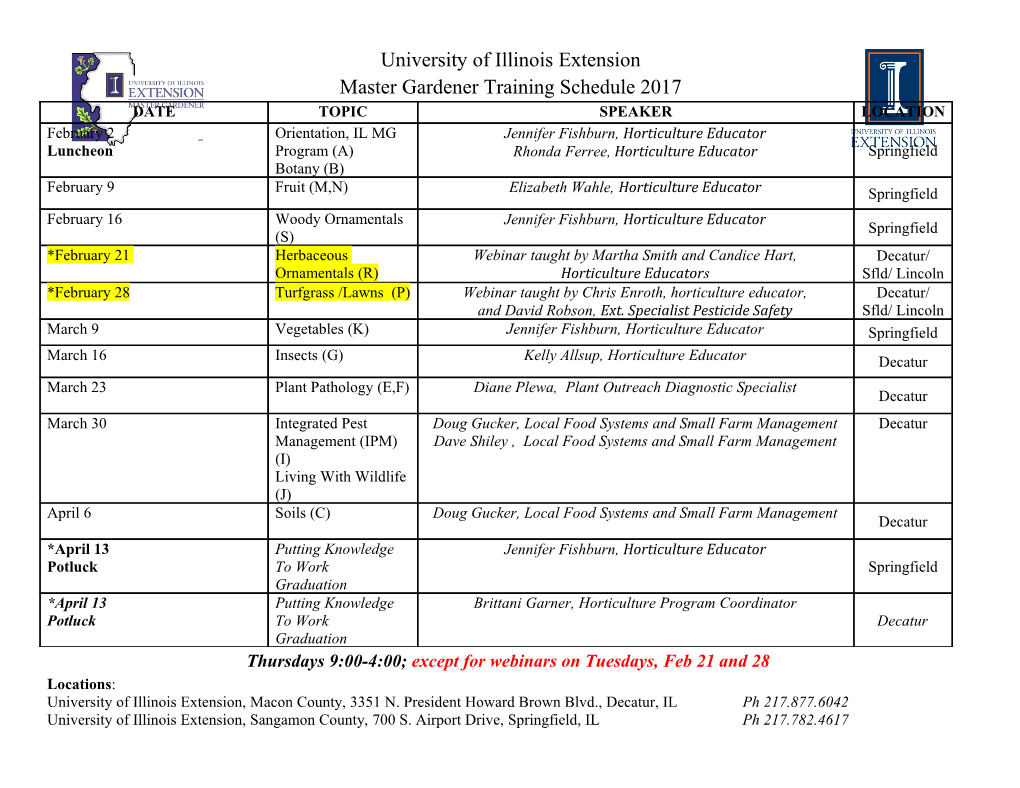
www.saltworkconsultants.com John Warren - Thursday Feb 10, 2019 VolatileSalty evaporite Matters interactions with magma, Part 1 of 3: Indications of hydrated salts? teractive and external to the magma). Both encompass outcomes Introduction that can include a variety of substantial ore deposits (Lebedev Direct and indirect interactions between magma and evaporites and Pinsky, 2017; Warren, 2016; Chapter 16; Morteani et al., at a regional scale are neither well documented, nor well un- 2013). Only in situations where igneous sills and dykes have in- derstood. Mostly, this is because little or no salt remains once truded salt masses, with contacts preserved, can direct effects of the high-temperature igneous-driven interactions have run their magma-salt interaction be documented. Even then, determining course; instead, there is a suite of indirect geochemical and in- the timing of the evaporite igneous interaction can be problemat- dicator-mineral assemblages (Warren, 2016; Morteani et al., ic; one must ask if the chemistry and texture indicate, 1) syn-ig- 2013). Aside from the presence of what can be ambiguous in- neous emplacement, or 2) post-emplacement alteration and dicator mineral suites, some hard-rock geologists with careers deeply circulating groundwater flushing, or 3) a combination. working in igneous and metamorphic terranes may not be well Historically, ignous melt interactions are considered in terms of versed in textures indicative of the former presence of sedimen- the dominant subsurface evaporite phases - halite and anhydrite tary evaporites, nor their varying volatility, nor their meta-evap- - both anhydrous salt minerals. In this article we will considered oritic and meta-igneous siblings. also igneous interactions with hydrous salts, like carnallite, ka- The term pyrometasomatic encompasses some, but not all, of the inite, polyhalite and gypsum; any of which can be locally signif- types of salt-magma interaction and reactions that occur when icant bed constituents in a halite-dominant basin fill (Table 1). evaporites and molten magmas of different types are nearby. Orthomagmatic and paramagmatic evaporite associations are Styles of evaporites interactions with magma are a spectrum, distinct from occurrences of primary igneous/magmatic anhy- with two endmember situations; 1) orthomagmatic (salt-assimi- drites, which precipitate from sulphate-saturated melts. Igneous lative and internal to the magma), and 2) paramagmatic (salt-in- anhydrite forms independently of any sedimentary evaporite Salt Formula Decomposition/Melt point (°C) assimilation, as seen, for example, in anhydrite crystals crystal- lised in trachyandesitic pumice erupted from El Chichón Volca- Halite NaCl 804 anhydrous melt no in 1982, or in dacitic pumices erupted from Mount Pinatubo Gypsum CaSO .2H O 100-150 loss of water 4 2 in 1991 and in acidic lavas in the Yanacocha district of northern Anhydrite CaSO 1460 anhydrous melt 4 Peru (Luhr et al., 2008; Chambefort et al., 2008). These evaporite Carnallite KMgCl3.6H2O 110-120 loss of water assimilations are also distinct from fumarolic anhydrite, which Sylvite KCl 750-790 anhydrous melt precipitates where groundwaters and sulphur-bearing magmatic fluids interact, as on Usu Volcano, Hokkaido, and many central Bischofite MgCl2.6H2O >118 loss of water 712 anhydrous melt American and Andean volcanoes such as El Laco (Zimbelman et al., 2005). Likewise, they are distinct from the anhydrite precip- Epsomite MgSO4.7H2O >70-80 loss of water itates (white smokers) in and below submarine vents across nu- Kieserite MgSO4.H2O 150-200 loss of water 1120-1150 anhydrous melt merous mid-oceanic ridges (Humphris et al., 1995). See Warren Polyhalite K Ca Mg(SO ) .2H O 230-280 loss of water 2016 (Chapter 16) for more geological detail on these non-evap- 2 2 4 4 2 orite-igneous anhydrite occurrences. Trona Na3H(CO3)2.2H2O >70 loss of water Nahcolite NaHCO 270 anhydrous melt 3 Cooking with salt (thermal decomposition TYPICAL MAGMA/ERUPTION TEMPERATURES of hydrated versus non-hydrated salts) Picritic 1400 -1500 Perhaps the most critical factor controlling the local intensity of magmatic interaction with an evaporitic country rock is whether Basaltic 1100-1200 or not the intruded sedimentary evaporite assemblage, in prox- Rhyolitic 800-900 imity to an igneous heat source, contains abundant hydrated Natrocarbonatite 500-600 salts, such as gypsum, polyhalite or carnallite. Hydrated evap- Table 1. Decomposition and melting points of selected salts and typical orite salts, when interacting with the igneous realm, are highly ranges of magma temperatures (after Warren, 2016). Page 1 www.saltworkconsultants.com volatile and likely to decompose. They 12 tend to release their water of crystalli- 200° sation at temperatures many hundreds 10 of degrees below the melting points of their anhydrous counterparts (Table 1). 8 250° In contrast, anhydrous salts, such as 6 halite beds intruded by igneous dykes 300° or sills, are much less reactive. At a Time (months) Time 4 350° local scale (measured in metres) with 400° respect to an intrasalt-igneous interac- 2 150° tion, there are a number of document- ed thermally-driven alteration styles, 0 1 2 3 4 5 typically created by the intrusion of Distance (m) into salt from edge of a 1.8m-wide basalt dyke dolerite dykes and sills into cooler ha- Figure 1. Temperature effects in salt across time (1 year) and distance (m) from the edge of a 1.8m-wide lite, or the outflow of extrusive igneous basalt intrusion into Zechstein halite in the Fulda region, Germany (after Knipping, 1989; Warren, 2016). flows over cooler halite beds (Knip- ping and Herrmann, 1985; Knipping, alteration halo extends up to twice the thickness of the dolerite 1989; Grishina et al, 1992, 1998; Gutsche, 1988; Steinmann et sill above the sill and almost the thickness of the sill below (Fig- al., 1999; Wall et al., 2010). Hot igneous material interacts with ure 1). somewhat cooler anhydrous salt masses, typically halite or an- Four inclusion type associations were found in bedded halite as hydrite, to create narrow but distinct heat and mobile fluid-re- a function of the ratio of the distance of the sample from the lease envelopes(Figure 1), also reflected in the resulting recrys- intrusion contact (d) to the thickness of the intrusion (h), i.e. d/h tallised inclusion-modified salt textures in a heat halo, centred (Figure 2). Chevron structures with aqueous inclusions progres- on the intrusive (Figure 2). sively disappear as d/h decreases; the disappearance of chevrons Based on studies of inclusion chemistry and homogenization occurs at greater distances above than below the intrusive sill. At temperatures in fluid inclusions in bedded halite near intrusives, d/h < 5 above the sill, a low-density CO2 vapour phase appears it seems that the extent of the influence of a dolerite sill or dyke in brine inclusions, at d/h < 2 H2S-bearing liquid-CO2 inclu- in bedded salt is marked by fluid (brine)-inclusion migration. sions appear, sometimes associated with carbonaceous material This is evidenced by the disappearance of chevron structures and orthorhombic S8, and for d/h < 0.9, CaCl2, CaCl2.KCl and and consequent formation of clear (sparry) recrystallised halite, nCaCl2.n MgCl2 solids occur in association with free water and with a new set of higher-temperature brine inclusions located liquid CO2 inclusions, with H2S, SCO, and Sg. The d/h values at intercrystal or polyhedral intersections. Such a migration en- marking the transitions outlined above are lower below the sills velope is documented in bedded Cambrian halites intruded by than above. The water content of the inclusions progressively end-Permian dolerite dykes in the Tunguska region of Siberia decreases on approaching the sills, whereas their CO2 content (Figure 2; Grishina et al., 1992). There, as a rule of thumb, an and density increase. Carnallite, sylvite and calcium chloride can occur as solid inclusions in the two associations nearest to the sill Inclusion associations resulting from chevron halite alteration and for d/h<2. Carnallite and sylvite inclusion migration/modication halite chevron driven by dolerite sill emplacement occur as daughter minerals in brine Unchanged I II III IV inclusions. The presence of carbon d/h Association I is characterized by carnallite-bearing brine inclusions with a dioxide is taken to indicate fluid cir- 7 CO vapour bubble in clear halite. 2 culation and dissolution/recrystalli- 6 Association II is characterized by various ratios of liquid CO and brine. 2 sation phenomena induced by the ba- 5 Association III is characterized by the following types of solid salt inclusions salt intrusions. The origin of carbon 4 and uid inclusions: dioxide is likely related to carbonate 3 1) Highly concentrated brine with a metastable solid phase CaCl2·6H2O; 2) Brine with several salt solids: CaCl ·nH O, 2MgCl · 12H O; 2 2 2 2 2 dissolution during magmatism (see 3) Liquid CO2 with solid CaCl2·6H2O; 4) Liquid CO + several salt solids: CaCl ·nH O, 2MgCl ·CaCl · 12H O (± brine); 1 2 2 2 2 2 2 Salty Matters, Oct 31, 2016). 5) Liquid CO2 without brine; 6) Solid salt hydrate; h 7) Anhydrous solid salt In some shallow locations, relatively rapid magma emplacement can lead 1 Association IV is characterized by single-phase brine inclusions and by very rare inclusions with a small CO phase in addition to the brine. In 20 out of 23 2 2 to linear breakout trends outlined by samples, the halite is not massive halite, but lls dissolution holes in 3 dolomite beds. Occurrence of these inclusions does not depend on the d/h phreatomagmatic or phreatic explo- ratio. sion craters. Such phreatic explosion craters have been imaged on the Tertiary seafloor horizons in parts Brine CO2 CO2 Chevron Recrystallised Dolerite vapour liquid halite halite sill of the North Sea (Figure 3; Wall et Figure 2. Occurrence of main fluid inclusion associations as a function of the d/h ratio; where d is al., 2010).
Details
-
File Typepdf
-
Upload Time-
-
Content LanguagesEnglish
-
Upload UserAnonymous/Not logged-in
-
File Pages9 Page
-
File Size-