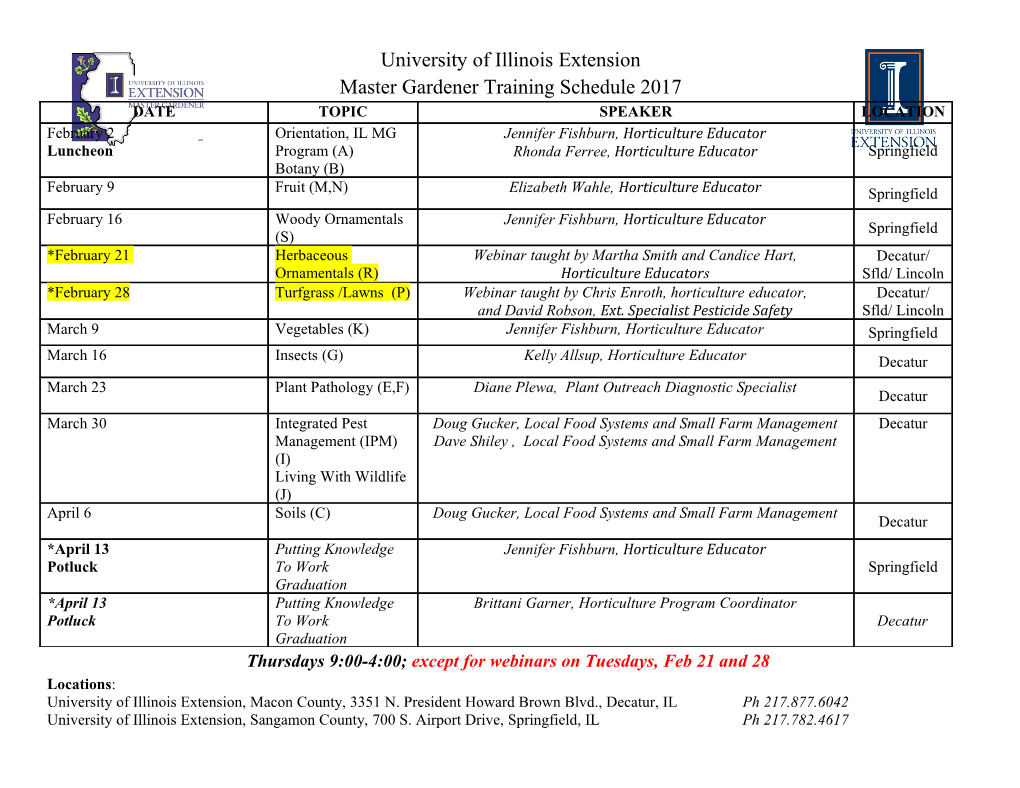
Effects of macroalgal mats on sediment nutrient release and benthic-pelagic coupling Jordan Stark, Skidmore College, Saratoga Springs, NY 12866 Abstract Nutrient loading, one of the most severe human impacts on coastal bays, leads to a range of ecological effects including the decline of seagrass beds and proliferation of macroalgal mats as well as other changes in nutrient cycling, oxygen levels and habitat quality. Here, I examined the effects of the presence of macroalgal mats on fine-scale nutrient and oxygen dynamics and benthic-pelagic coupling in a controlled lab setting. Decomposition rates were high, leading to production of sulfides and high variability in many measures. However, oxygen was produced at the surface of the macroalgal mats, indicating that photosynthesis did occur. N:P ratios varied from 0.5 in the thick mat treatment to 36.7 in the no mat treatment, indicating strong N limitation in the presence of macroalgal mats and some P limitation in their absence. Over the course of five days, chlorophyll a concentrations were 21.8±2.0 μg/L in the no mat (control) treatment but only 0.2±0.08 μg/L in the thick mat treatment, even though inorganic nitrogen and phosphorus were both available. This study demonstrates clear ecological effects of macroalgal mats on nutrient stoichiometry and hints at non-nutrient mechanisms of benthic-pelagic decoupling in the presence of macroalgae. Key Words Macroalgal mats; nutrient cycling; decomposition; eutrophication; benthic-pelagic coupling Introduction Marine systems are generally limited by nitrogen and sensitive to human nitrogen inputs (Vitousek and Howarth 1991). This is particularly evident in coastal systems, where productivity is high and human inputs of nutrients can affect ecosystem structure and function. On Cape Cod, nitrogen loading is particularly high due to nutrient inputs from wastewater and fertilizers (Valiela et al. 1992). Nutrient loading has been identified at the major stressor to Waquoit Bay, a shallow estuary in Falmouth and Mashpee, MA, and other area estuaries, leading to a wide range of problems including anoxic events, loss of fish habitat and changes to the food web (Serveiss et al. 2004, Valiela et al. 1992, D'Avanzo and Kremer 1994). One of the most noticeable effects of eutrophication is the replacement of high-quality habitat seagrass beds with thick mats of macroalgae which may reduce light levels and crowd out seagrasses (Hauxwell et al. 2003, McGlathery 2001, Valiela et al. 1997). In some systems, these mats can be more than 50 cm thick during blooms, making them the major primary producers and causing daily fluctuations in respiration and nutrient uptake (Krause-Jensen et al. 1999, Valiela et al. 1992). It is not clear how such thick accumulations of macroalgae can persist, since light is extinguished in the top few cm of the mat and decomposition occurs rapidly below the light layer (Peckol and Rivers 1996). When mats of macroalgae accumulate, nitrogen limitation may become more severe due to the low C:N ratio of macroalgae (~6:1) when compared to seagrasses (~20:1) (Duarte 1990). This can reduce phytoplankton biomass, as phytoplankton are heavily reliant on recycled nitrogen from the sediments that may be intercepted by algal mats. Here, I examine how macroalgal mats take up nutrients and alter nutrient cycling at the sediment-water interface using a novel system for sampling water at specific depths within macroalgal mats incubated in the lab. In addition to taking up nutrients, the respiration and photosynthesis of thick mats of macroalgae create fluctuations in oxygen concentrations over the day-night cycle. Photosynthesis is high at the surface of the mat, so oxygen levels can become supersaturated with oxygen trapped in the physical structure of the mat (Herbert 1999, Krause-Jensen et al. 1996). However, respiration levels are also high, so anoxic events may occur when light levels are insufficient to sustain high photosynthesis rates. In Waquoit Bay, anoxic events have occurred during periods of low light and high temperatures, leading to fish kills, while hypoxia occurs on a nearly daily basis at night during the summer (D'Avanzo and Kremer 1994). Light cannot penetrate to the bottom of thick algal mats, so in undisturbed areas rapid decomposition also occurs, increasing oxygen demand (Peckol and Rivers 1996). In addition to effects on animals, nitrifying and denitrifying bacteria may be impacted by nutrient demand and steep gradients in oxygen caused by macroalgal photosynthesis and respiration. Ammonia released by decomposition of organic matter in sediments may be converted to nitrate under aerobic conditions by chemoautotrophic nitrifying bacteria that fix carbon using energy from the oxidation of ammonium. Conditions that promote nitrification (high oxygen and potentially high ammonium levels) occur primarily in the photosynthetically active layer of the algal mat. The nitrate produced by these bacteria can then diffuse into the anaerobic zones of the algal mat (below the light layer) where it can be used as an electron acceptor by denitrifying bacteria. These two processes may be coupled, removing ammonium from the system by first nitrifying and then denitrifying it. Photosynthetically active macroalgal mats can either increase coupled nitrification/denitrification if oxygen is limiting for the nitrifying bacteria or decrease coupled nitrification/denitrification rates if the macroalgae outcompete the bacteria for ammonium or nitrate (Dalsgaard 2003, Krause-Jensen et al. 1999). Depending on the overall effect on denitrification, macroalgal mats may worsen or alleviate some human nutrient loading to coastal systems where they occur. For this study, I artificially isolated macroalgal mats in mesocosms with sediment to test the effects of macroalgal mat presence and thickness on nutrient uptake, oxygen fluctuations, denitrification rates and benthic-pelagic coupling. I also examined the decomposition rate of the mats using three different models. The physical structure of macroalgal mats is an important factor in their effects on ecosystems, so I created sampling devices with permanently installed tubes at various depths within the macroalgal mats to allow sampling without disturbing the mats. I then sampled these over daily and weekly time periods to test three hypotheses: 1. Greater densities of macroalgae increase fluctuation in oxygen levels temporally and spatially. a. High oxygen should be present near the surface of the mat and during the day, while anoxia should occur at night and at the base of the mat where light does not penetrate. 2. Macroalgae take up a significant fraction of ammonium and phosphate released from sediments, decreasing nutrient levels in the overlying water. a. Temporal and spatial patterns in nutrient concentrations should occur, including nutrient uptake by macroalgae and trapping of nutrients within the physical structure of macroalgal mats. b. Macroalgal mats should reduce phytoplankton biomass in the overlying water. 3. Coupled nitrification and denitrification will change based on the interaction between nutrient levels and oxygen levels in two possible ways. a. Nitrification should produce high nitrate levels where oxygen is present at the surface of the mat, while denitrification should reduce nitrate levels and produce N2 gas near the base of the mat. b. If macroalgae outcompete bacteria for nitrogen, nitrification and denitrification will decrease with increased macroalgal presence. Methods Mesocosm preparation and maintenance On 11 November, I collected macroalgae and sediment from the mouth of the Child’s River where it enters Waquoit Bay in Falmouth, MA. I collected the sediment from near the edge of the water at low tide and macroalgae from slightly further up the Child’s. The macroalgae was in mats about 10 cm thick with a mixture of Gracilaria and Ulva, which I collected in the proportion that they occurred in the field (about 80% Gracilaria, 20% Ulva). I brought the algae and sediment back to the lab, where I sorted through it to remove animals, including snails, sea cucumbers and grass shrimp. I then stored this macroalgae in a slow flow- through tank with running seawater until I established my mesocosms. On 12 November, I sieved the sediment with a 4 mm coarse sieve to remove animals and large pieces of plants and homogenized all collected sediment to ensure even distribution of sediment among the tanks. On 13 November, I began establishing the mesocosms. First, I placed sampling devices made from PVC and plastic tubing into each of six clear fiberglass mesocosms (30 cm diameter). Each sampling device had tubes running from specified depths to the surface of the mesocosm to allow me to take samples with minimal disturbance to the macroalgal mat (Figure 1, Table 1). These tubes each had a volume of 15 mL. I then added sieved sediment to about 10 cm above the base of the mesocosms and gently filled them with seawater to 35 cm above the sediment. On 14 November, I added 10 cm of the mixed macroalgae to the thin mat treatment tanks (n=2) and 40 cm of macroalgae to the thick mat treatment tanks (n=2). I did not add any algae to the sediment only treatment tanks (n=2). I then filled all tanks with seawater to 70 cm above the sediment (total volume=51 L water) and filled a larger tank surrounding all six experimental tanks with water to 40 cm to buffer temperature changes. At this time, I established a 12 hour day/night cycle with a growth light suspended over the tanks and placed an air stone in the overlying water of each tank (about 20 cm into the water) to keep it oxygenated. On 19 November, I wrapped black plastic around the mesocosms with macroalgae to the height of the macroalgal mat to reduce light entering the mat from the sides. After establishment, I replaced about 15% of the water in each tank on 16 and 19 November with fresh seawater.
Details
-
File Typepdf
-
Upload Time-
-
Content LanguagesEnglish
-
Upload UserAnonymous/Not logged-in
-
File Pages38 Page
-
File Size-