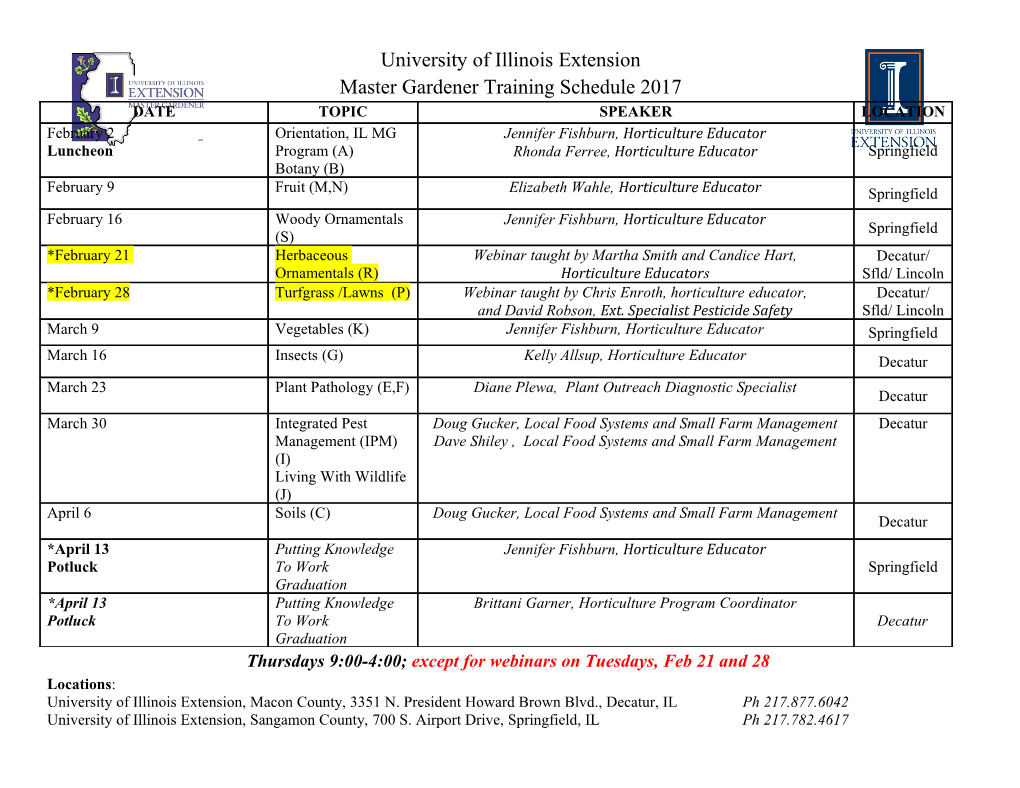
Chapter 3: Radioactivity Radioactivity from Direct Excitation X-ray Emission 163 NPRE 441, Principles of Radiation Protection An Overview of Radiation Exposure to US Population 164 NPRE 441, Principles of Radiation Protection Chapter 3: Radioactivity X-ray Imaging Examples 165 NPRE 441, Principles of Radiation Protection Chapter 3: Radioactivity X-ray Generation – X-ray Tube Andrew Webb, Introduction to Biomedical Imaging, 2003, Wiley- Interscience. Motor, Why? Filament Rotating target Electron beam? How are electrons generated? 166 NPRE 441, Principles of Radiation Protection Chapter 3: Radioactivity X-ray Generation – Bremsstrahlung • Target nucleus positive charge (Z·p+) attracts incident e- • Deceleration of an incident e- occurs in the proximity of the target atom nucleus • Energy lost by e- is gained by the EM photon (x-ray) generated • The impact parameter distance, the closest approach to the nucleus by the e- determines the amount of E loss • The Coulomb force of attraction varies strongly with distance (µ 1/r2); ↓ distance → ↑ deceleration and E loss → ↑ photon E • Direct impact on the nucleus determines the maximum x-ray E (Emax) 167 NPRE 441, Principles of Radiation Protection Chapter 3: Radioactivity X-ray Generation – Bremsstrahlung Interestingly, this process creates a relatively uniform spectrum. Intensity = nhu e0 Photon energy spectrum 168 NPRE 441, Principles of Radiation Protection Chapter 3: Radioactivity The Unfiltered Bremsstrahlung Spectrum Thick Target X-ray Formation We can model target as a series of thin targets. Electrons successively loses energy as they moves deeper into the target. Electrons X-rays Relative Intensity e0 Each layer produces a flat energy spectrum with decreasing peak energy level. 169 NPRE 441, Principles of Radiation Protection Chapter 3: Radioactivity X-ray Generation – Characteristic X-rays • e- of the target atom have a binding energy (BE)that depends on atomic Z 2 (rem: BEKµ Z ) and the shell (BEK> BEL> BEM> ... ) • When e-(KE) incident on the target exceeds the target atom e-(BE), it’s energetically possible for a collisional interaction to eject the bound electron and ionize the atom. • What would happen then? 170 NPRE 441, Principles of Radiation Protection Chapter 3: Radioactivity X-ray Generation – X-ray Tube Andrew Webb, Introduction to Biomedical Imaging, 2003, Wiley- Interscience. Motor, Why? Filament Rotating target Electron beam? How are electrons generated? 171 NPRE 441, Principles of Radiation Protection Chapter 3: Radioactivity X-ray Generation – Characteristic X-rays • Within each shell (other than K) there are discrete E orbitals (ℓ = 0, 1, ... , n-1) → characteristic x-ray fine E splitting • Characteristic x-rays other than those generated through K-shell transitions are unimportant An experimentally measured X- ray energy spectrum from a micro-focus X-ray source 172 NPRE 441, Principles of Radiation Protection Chapter 3: Radioactivity X-ray Generation – Characteristic X-rays Superimposed multiple flat spectrum with decreasing cutoff energy Low energy X-rays suffer attenuation inside the anode Further attenuation by the source package. External filtering to reduce low E photons à lower does Beam hardening 173 NPRE 441, Principles of Radiation Protection Chapter 3: Radioactivity X-ray Generation – X-ray Tube 174 NPRE 441, Principles of Radiation Protection Chapter 3: Radioactivity X-ray Generation – Bremmstrahlung • Target nucleus positive charge (Z·p+) attracts incident e- • Deceleration of an incident e- occurs in the proximity of the target atom nucleus • Energy lost by e- is gained by the EM photon (x-ray) generated • The impact parameter distance, the closest approach to the nucleus by the e- determines the amount of E loss • The Coulomb force of attraction varies strongly with distance (µ 1/r2); ↓ distance → ↑ deceleration and E loss → ↑ photon E • Direct impact on the nucleus determines the maximum x-ray E (Emax) 175 NPRE 441, Principles of Radiation Protection Sources of Electromagnetic Radiation – Synchrotron Radiation Lorentz force: Fm = q (v x B) 176 NPRE 441, Principles of Radiation Protection Sources of Electromagnetic Radiation – Synchrotron Radiation 177 NPRE 441, Principles of Radiation Protection Sources of Electromagnetic Radiation – Synchrotron Radiation 178 NPRE 441, Principles of Radiation Protection Chapter 3: Radioactivity X-ray emission is typically associated with other types of radiations, which should be considered for shielding design or dose assessment. 179 NPRE 441, Principles of Radiation Protection Typical Decay Products from Unstable Radioisotopes Alpha decay Beta decay Beta decay Beta-plus decay Orbital electron capture (E.C.) Alpha particles Daughter nuclei Beta particles Positrons Daughter nuclei Annihilation Bremsstrahlung gamma rays X-rays Excited Daughter Nuclei Gamma ray emission Internal conversion (I.C.) IC electrons Excited atoms Gamma rays Auger electrons Characteristic Bremsstrahlung X-rays X-rays 180 Staining of Tissue sections… Bremsstrahlung X- rays also serve as source of radiation dose beta particles getting out and irridiating the hand 181 Neutron Sources 182 NPRE 441, Principles of Radiation Protection Typical Scenarios Involving Neutron Radiation • Portable neutron sources • Nuclear reactors • Medical applications 183 NPRE 441, Principles of Radiation Protection Neutron Sources – Spontaneous Fission Cf-252 neutron source can be made An engineer tests the prototype Timed Neutron Detector, a device that detects landmines. The neutron source of the landmine extremely compact detector holds a tiny amount of californium-252. (Photo credit: Pacific Northwest National Lab) 184 NPRE 441, Principles of Radiation Protection Active Interrogation for Finding Special Nuclear Materials Mobile Dual Neutron/Gamma Interrogation System 185 Inside a Nuclear Reactor 186 Nuclear Fission from Slow Neutrons and Water Moderator 187 Chapter 4: Interaction of Radiation with Matter – Interaction of Neutrons with Matter Neutron Induced Reactions 1 10 7 4 0 n+ 5B®3 Li+2 He F Cross section for thermal neutron is 3840 barns. F Q=2.31MeV when the daughter nucleus is in an excited state (93%) and 2.79MeV when the Li nucleus is in ground state (7%). F Widely used for thermal neutron detection. BF3 is a gas that can be used directly in a neutron counter. Boron is also used as a liner inside the tubes of proportional counters for neutron detection. 188 NPRE 441, Principles of Radiation Protection, Spring 2020 Proton Therapy http://www.floridaproton.org/what-is-proton-therapy/benefits The downside of this strategy is that proton interactions with materials in the beamline will create high-energy secondary neutrons. The high linear energy transfer (LET) of neutrons makes them extremely efficient at ionization, and far more likely to cause cell death than low-LET particles, such as X-rays or protons. 189 Thermal Neutron Capture Radiation Therapy Fig.1 boron neutron capture therapy (BNCT) can be performed at a facility with a nuclear reactor or at hospitals that have developed alternative neutron sources. A beam of epithermal neutrons penetrates the brain tissue, reaching the malignancy. Once there the epithermal neutrons slow down and these low-energy neutrons combine with boron-10 (delivered beforehand to the cancer cells by drugs or antibodies) to form boron-11, releasing lethal radiation (alpha particles and lithium ions) that can kill the tumor.[1] Boron neutron capture therapy Barth, Rolf F.; Soloway, Albert H.; Fairchild, Ralph G. (1990). "Boron Neutron Capture Therapy for Cancer". Scientific American 263 (4): Figure from: http://en.wikipedia.org/wiki/File:NeutronCaptureTherapyImage.jpg 100–3, 106–7. Bibcode:1990SciAm.263d.100B. doi:10.1038/scientificamerican1090-100. PMID 2173134.190 Neutrons Sources 191 NPRE 441, Principles of Radiation Protection Neutron Sources – Spontaneous Fission Cf-252 neutron source can be made An engineer tests the prototype Timed Neutron Detector, a device that detects landmines. The neutron source of the landmine extremely compact detector holds a tiny amount of californium-252. (Photo credit: Pacific Northwest National Lab) 192 NPRE 441, Principles of Radiation Protection 193 NPRE 441, Principles of Radiation Protection Neutron Sources – Spontaneous Fission Spontaneous fission of tarnsuranic heavy nuclides, such as 252Cf, produces several fast neutrons, in addition to heavy fission products, prompt fission gamma rays and beta and gamma ray activities. • Half-life: 2.65 years • Neutron yield: 0.116n/s per Bq, or 2.3×106 n/s per mg • Neutron energy peaking at 0.5MeV and extends beyond 10MeV. Measured neutron energy spectrum from spontaneous fission of 252Cf Page 19-27, Radiation Detection and Measurements, Third Edition, G. F. Knoll, John Wiley & Sons, 1999. 194 NPRE 441, Principles of Radiation Protection Neutron Sources – Radioisotope (a,n) Sources Energetic alpha particles can induce (a,n) reaction in certain target materials. 4 9 12 1 2a +4 BeÞ 6 C+0 n Q - value : 5.71MeV The source is normally prepared in the form of alloy (MBe13), where M is alpha-emitting radioisotopes A practical neutron source Page 19-27, Radiation Detection and Measurements, Third Edition, G. F. Knoll, John Wiley & Sons, 1999. 195 NPRE 441, Principles of Radiation Protection Neutron Sources – Radioisotope (a,n) Sources James Tuner, Atoms, radiation and Radiation Protection, p210-p211. 196 NPRE 441, Principles of Radiation Protection Neutron Sources – Radioisotope (a,n) Sources A typical neutron energy spectrum from an 239Pu/Be
Details
-
File Typepdf
-
Upload Time-
-
Content LanguagesEnglish
-
Upload UserAnonymous/Not logged-in
-
File Pages59 Page
-
File Size-