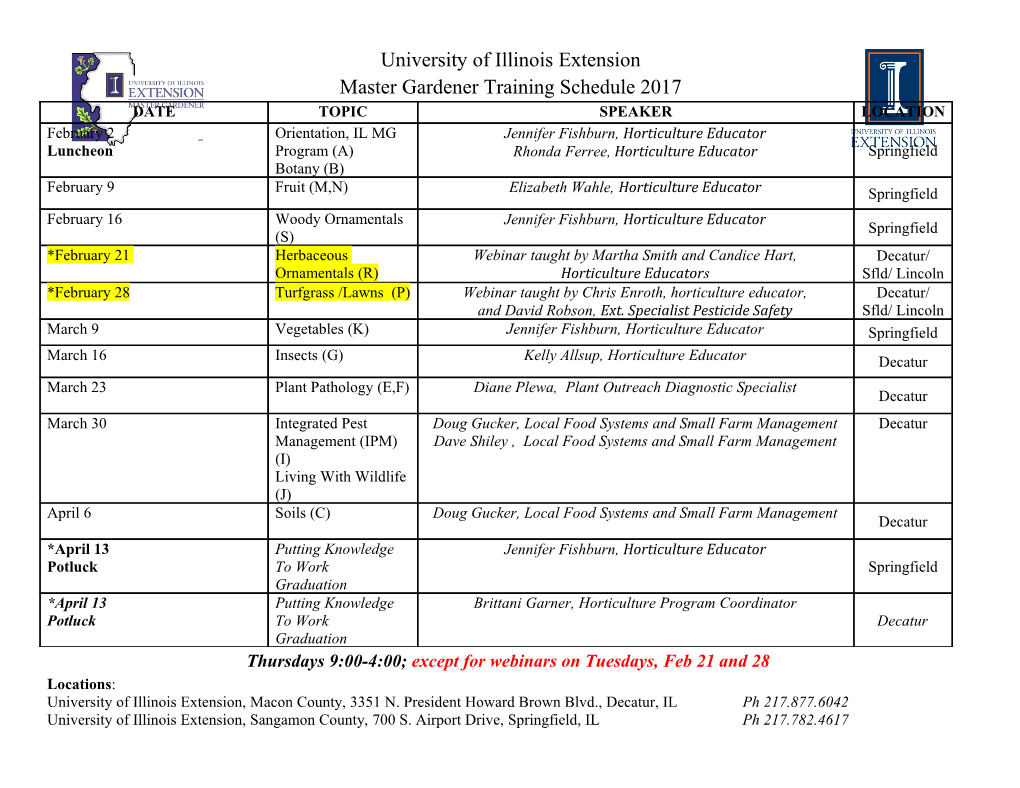
Electrophilic Aromatic Substitution 18 18.1 Electrophilic aromatic substitution 18.2 The general mechanism 18.3 Halogenation 18.4 Nitration and sulfonation 18.5 Friedel–Crafts alkylation and Friedel–Crafts acylation 18.6 Substituted benzenes 18.7 Electrophilic aromatic substitution of substituted benzenes 18.8 Why substituents activate or deactivate a benzene ring 18.9 Orientation effects in substituted benzenes 18.10 Limitations on electrophilic substitution reactions with substituted benzenes 18.11 Disubstituted benzenes 18.12 Synthesis of benzene derivatives 18.13 Halogenation of alkyl benzenes 18.14 Oxidation and reduction of substituted benzenes 18.15 Multistep synthesis LSD, commonly referred to as “acid,” is a powerful hallucinogen prepared from lysergic acid, the principal organic compound derived from one of the ergot fungi. Immortalized in the 1967 Beatles’ song, “Lucy in the Sky with Diamonds,” LSD produces sensory illusions, making it diffi cult for the user to distinguish between reality and fantasy. Given its potent biological properties, LSD has been the target of several different laboratory syntheses. A key step in one of them involves carbon–carbon bond formation using electrophilic aromatic substitution, the most common reaction of aromatic compounds and the subject of Chapter 18. 641 smi75625_641-687ch18.indd 641 11/5/09 11:48:21 AM 642 Chapter 18 Electrophilic Aromatic Substitution Chapter 18 discusses the chemical reactions of benzene and other aromatic com- pounds. Although aromatic rings are unusually stable, making benzene unreactive in most of the reactions discussed so far, benzene acts as a nucleophile with certain electrophiles, yielding substitution products with an intact aromatic ring. We begin with the basic features and mechanism of electrophilic aromatic substitution (Sec- tions 18.1–18.5), the basic reaction of benzene. Next, we discuss the electrophilic aromatic sub- stitution of substituted benzenes (Sections 18.6–18.12), and conclude with other useful reactions of benzene derivatives (Sections 18.13–18.14). The ability to interconvert resonance structures and evaluate their relative stabilities is crucial to understanding this material. 18.1 Electrophilic Aromatic Substitution Based on its structure and properties, what kinds of reactions should benzene undergo? Are any of its bonds particularly weak? Does it have electron-rich or electron-defi cient atoms? • Benzene has six o electrons delocalized in six p orbitals that overlap above and below the plane of the ring. These loosely held o electrons make the benzene ring electron rich, and so it reacts with electrophiles. • Because benzene’s six o electrons satisfy Hückel’s rule, benzene is especially stable. Reactions that keep the aromatic ring intact are therefore favored. As a result, the characteristic reaction of benzene is electrophilic aromatic substitution—a hydrogen atom is replaced by an electrophile. H E Electrophilic aromatic + + substitution + E + H electrophile substitution of H by E Benzene does not undergo addition reactions like other unsaturated hydrocarbons, because addi- tion would yield a product that is not aromatic. Substitution of a hydrogen, on the other hand, keeps the aromatic ring intact. H H X X2 Addition The product is not aromatic. H X H H E E+ Substitution The product is aromatic. Five specifi c examples of electrophilic aromatic substitution are shown in Figure 18.1. The basic mechanism, discussed in Section 18.2, is the same in all fi ve cases. The reactions differ only in the identity of the electrophile, E+. Problem 18.1 Why is benzene less reactive towards electrophiles than an alkene, even though it has more π electrons than an alkene (six versus two)? 18.2 The General Mechanism No matter what electrophile is used, all electrophilic aromatic substitution reactions occur via a two-step mechanism: addition of the electrophile E+ to form a resonance-stabilized carbocation, followed by deprotonation with base, as shown in Mechanism 18.1. smi75625_641-687ch18.indd 642 11/5/09 11:48:22 AM 18.2 The General Mechanism 643 Figure 18.1 Reaction Electrophile Five examples of electrophilic [1] Halogenation—Replacement of H by X (Cl or Br) aromatic substitution H X + + + X2 E = Cl or Br FeX3 X = Cl aryl halide X = Br [2] Nitration—Replacement of H by NO2 H NO2 + HNO + 3 E = NO2 H2SO4 nitrobenzene [3] Sulfonation—Replacement of H by SO3H H SO H 3 + SO + 3 E = SO3H H2SO4 benzenesulfonic acid [4] Friedel–Crafts alkylation—Replacement of H by R H R RCl E+ = R+ AlCl3 alkyl benzene (arene) Friedel–Crafts alkylation and [5] Friedel–Crafts acylation—Replacement of H by RCO acylation, named for Charles Friedel and James Crafts who O discovered the reactions in the H C R + nineteenth century, form new RCOCl E+ = RCO carbon–carbon bonds. AlCl3 ketone Mechanism 18.1 General Mechanism—Electrophilic Aromatic Substitution Step [1] Addition of the electrophile (E+) to form a carbocation + H H H • Addition of the electrophile (E ) forms a new C – E bond H + E E E using two π electrons from the benzene ring, and E+ + + generating a carbocation. This carbocation intermediate is not aromatic, but it is resonance stabilized—three resonance-stabilized carbocation resonance structures can be drawn. • Step [1] is rate-determining because the aromaticity of the benzene ring is lost. Step [2] Loss of a proton to re-form the aromatic ring H • In Step [2], a base (B:) removes the proton from the B E E carbon bearing the electrophile, thus re-forming the + + + HB aromatic ring. This step is fast because the aromaticity of the benzene ring is restored. • Any of the three resonance structures of the carbocation intermediate can be used to draw the product. The choice of resonance structure affects how curved arrows are drawn, but not the identity of the product. smi75625_641-687ch18.indd 643 11/5/09 11:48:22 AM 644 Chapter 18 Electrophilic Aromatic Substitution The fi rst step in electrophilic aromatic substitution forms a carbocation, for which three reso- nance structures can be drawn. To help keep track of the location of the positive charge: • Always draw in the H atom on the carbon bonded to E. This serves as a reminder that it is the only sp3 hybridized carbon in the carbocation intermediate. • Notice that the positive charge in a given resonance structure is always located ortho or para to the new C – E bond. In the hybrid, therefore, the charge is delocalized over three atoms of the ring. Always draw in the H atom at the site of electrophilic attack. + H H H H δ + E E E E + + δ+ δ+ (+) ortho to E (+) para to E (+) ortho to E hybrid This two-step mechanism for electrophilic aromatic substitution applies to all of the electrophiles in Figure 18.1. The net result of addition of an electrophile (E+) followed by elimination of a proton (H+) is substitution of E for H. The energy changes in electrophilic aromatic substitution are shown in Figure 18.2. The mecha- nism consists of two steps, so the energy diagram has two energy barriers. Because the fi rst step is rate-determining, its transition state is higher in energy. Problem 18.2 In Step [2] of Mechanism 18.1, loss of a proton to form the substitution product was drawn using one resonance structure only. Use curved arrows to show how the other two resonance structures can be converted to the substitution product (PhE) by removal of a proton with :B. 18.3 Halogenation The general mechanism outlined in Mechanism 18.1 can now be applied to each of the fi ve spe- cifi c examples of electrophilic aromatic substitution shown in Figure 18.1. For each mechanism we must learn how to generate a specifi c electrophile. This step is different with each electro- Figure 18.2 Energy diagram for transition state electrophilic aromatic Step [1] substitution: transition state + + Step [2] PhH + E → PhE + H Ea[2] E [1] a δ+ H Energy E δ+ δ+ H E + + E + H+ Reaction coordinate • The mechanism has two steps, so there are two energy barriers. • Step [1] is rate-determining; its transition state is at higher energy. smi75625_641-687ch18.indd 644 11/5/09 11:48:23 AM 18.3 Halogenation 645 phile. Then, the electrophile reacts with benzene by the two-step process of Mechanism 18.1. These two steps are the same for all fi ve reactions. In halogenation, benzene reacts with Cl2 or Br2 in the presence of a Lewis acid catalyst, such as FeCl3 or FeBr3, to give the aryl halides chlorobenzene or bromobenzene, respectively. Analo- gous reactions with I2 and F2 are not synthetically useful because I2 is too unreactive and F2 reacts too violently. H Cl Cl2 Chlorination FeCl3 chlorobenzene H Br Br2 Bromination FeBr3 bromobenzene In bromination (Mechanism 18.2), the Lewis acid FeBr3 reacts with Br2 to form a Lewis acid– base complex that weakens and polarizes the Br – Br bond, making it more electrophilic. This reaction is Step [1] of the mechanism for the bromination of benzene. The remaining two steps follow directly from the general mechanism for electrophilic aromatic substitution: addition of the electrophile (Br+ in this case) forms a resonance-stabilized carbocation, and loss of a proton regenerates the aromatic ring. Mechanism 18.2 Bromination of Benzene Step [1] Generation of the electrophile + – • Lewis acid–base reaction of Br2 with FeBr3 Br Br + FeBr3 Br Br FeBr3 forms a species with a weakened and Lewis base Lewis acid electrophile polarized Br – Br bond. This adduct serves (serves as a source of Br+) as a source of Br+ in the next step. Step [2] Addition of the electrophile to form a carbocation H H H • Addition of the electrophile forms a new H + + – Br Br Br C – Br bond and generates a carbocation.
Details
-
File Typepdf
-
Upload Time-
-
Content LanguagesEnglish
-
Upload UserAnonymous/Not logged-in
-
File Pages47 Page
-
File Size-