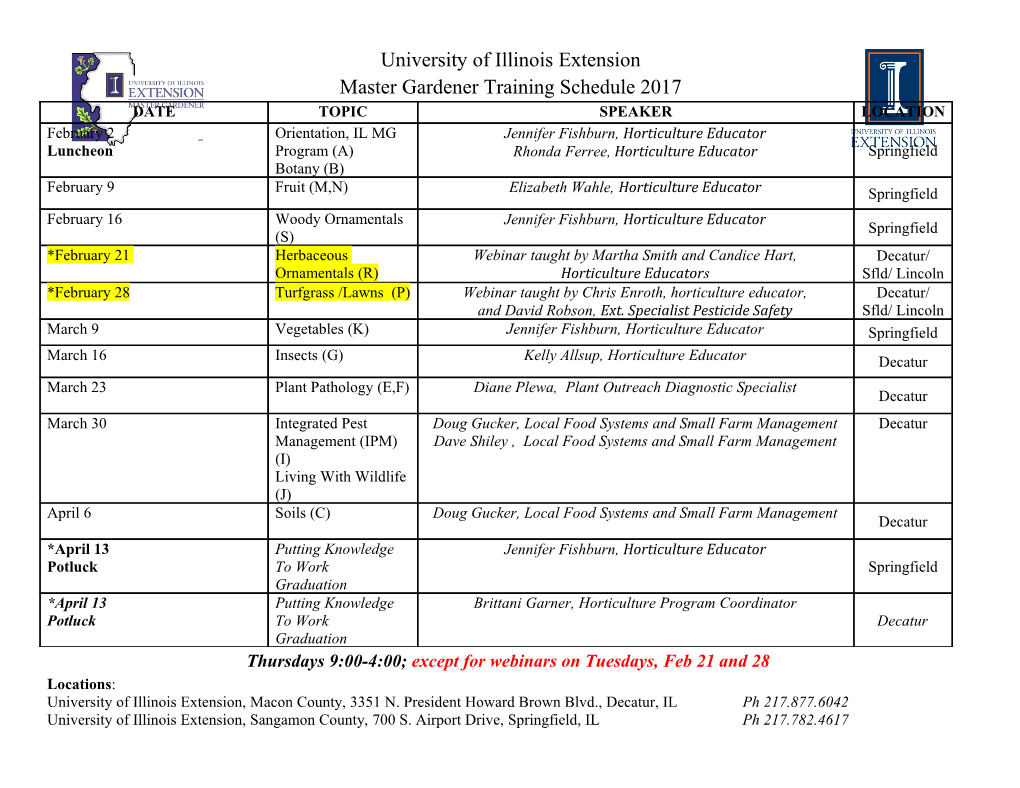
C H N 129.0 10 11 128.0 127.3 29.2 38.9 11.4 CDCl 135.8 120.7 3 TMS 1.92 3.72 (2H, dq, J=7.4, 7.1) (1H, t, J=7.1) 7.3 1.06 (5H, m) (3H, t, J=7.4) 187 Chapter 20: Enols and Enolates O E+ O H Enolate O E carbonyl H O E+ Enol 20.1: Enol Content and Enolization O β' β γ' α' α γ 188 94 Tautomers: isomers, usually related by a proton transfer, that are in equilibrium. Keto-enol tautomeric equilibrium lies heavily in favor of the keto form (see Table 20.1, p. 821). H O O C C H C C enol keto C=C ΔH° = 611 KJ/mol! C=O ΔH° = 735 KJ/mol! C-O 380! C-C 370! O-H 426! C-H 400! ΔH° = -88 KJ/mol! OH O C H H3C C H3C CH3 H 99.9999999 % 0.0000001% 189 Enolization is acid- and base-catalyzed (p. 823): Base-catalyzed mechanism: Acid-catalyzed mechanism: 190 95 α-Halogenation of Aldehydes and Ketones - α-proton of aldehydes and ketones can be replaced with a -Cl, -Br, or -I (-X) with Cl2 , Br2 , or I2 , (X2) respectively. The reaction proceeds through an enol. O O H X2, H-X X H H + H-X X= Cl, Br, I Rate= k [ketone/aldehyde] [H+] rate dependent on enol formation and not [X2] α,β-unsaturated ketones and aldehydes: α -bromination followed by elimination O O O CH3 CH - + 3 Br2, CH3CO2H (H3C)3CO K CH3 Br E2 Why is one enol favored over the other? OH O OH H+ CH3 CH3 CH3 191 Hell-Volhard-Zelinsky Reaction – α-halogenation of carboxylic acids: O O Br2, PBr3 H C Br C C OH C OH then H2O Mechanism of α-halogenation goes through an acid bromide intermediate. An acid bromide enolizes more readily than a carboxylic acid. Mechanism is analogous to the α-halogenation of aldehydes and ketones The α-halo carboxylic acid can undergo substitution to give α-hydroxy and α -amino acids. Br O O K2CO3, H2O HO R C C OH R C C OH Δ H H O Br H N O NH3, H2O 2 192 R C C OH R C C OH H H 96 20.2: Enolates Typical pKa’s of the α-protons carbonyl compounds (Table 20.1, p. 825): aldehydes 17 O O O C C C ketones 19 H3C H H3C CH3 H3C OCH3 esters 25 O amides 30 C H3C C N H C N(CH ) nitriles 25 3 3 2 Acidity of 1,3-dicarbonyl compounds O O C C H3C OCH3 H3C CH3 esterester! ketone ppKaa == 242254! pKa= 19 O O O O O O C C C C C C H3C C OCH3 H C C CH H3CO C OCH3 3 3 H H H H H H 1,3-diester 1,3-keto ester 1,3-diketone 193 pKa= 13 pKa= 11 pKa= 9 The inductive effect of the carbonyl causes the α-protons to be more acidic. The negative charge of the enolate ion (the conjugate base of the carbonyl compound) is stabilized by resonance delocalization. The pKa of the α-protons of aldehydes and ketones is in the range of 16-20 resonance effect δ - inductive O O δ+ O + B effect C H C C + H-B δ+ C C δ+ C carbonyl Enolate anion H C C C C C C O O O O H3C CH3 C H3CH2COH H3C CH3 ethane acetone ethanol 194 pKa= 50-60 pKa= 19 pKa= 16 97 O O O O O C C Cl C C C H3C CH3 H3C C H3C C H3C C CH3 H H H H H H pKa 19 14 16 9 O O + H O C + H3O C 2 H C CH H3C CH3 3 2 acid base conjugate conjugate base acid pKa 19 -1.7 (weaker acid) (weaker base) (stronger base) (stronger acid) O O + HO C + H O C H C CH 2 H3C CH3 3 2 acid base conjugate conjugate base acid pKa 19 15.7 (weaker acid) (weaker base) (stronger base) (stronger acid) _ O O O O C C + H2O C C + HO H C C CH H C C CH 3 3 3 3 H H H acid base conjugate conjugate base acid pKa 9 15.7 195 (stronger acid) (stronger base) (weaker base) (weaker acid) Lithium diisopropylamide (LDA): a super strong base + H CH CH CH C N H + H3CH2CH2CH2C Li N Li 3 2 2 3 diisopropylamine LDA pKa= 36 pKa= 60 O O C + N Li C + N H H3C CH3 H3C CH2 pKa= 19 pKa= 36 (stronger acid) (stronger base) (weaker base) (weaker acid) O O C + N C + N H H3C OCH2CH3 H2C OCH2CH3 pKa= 25 pKa= 36 (stronger acid) (stronger base) (weaker base) (weaker acid) 196 98 Enolate Regiochemistry – deprotonation of unsymmetrical ketones. O O O B: B: H3C H H3C H H3C H H H H H B-H B-H More substituted enolate: Less substituted enolate: thermodynamically more stable less hindered, formed faster kinetically The more substituted (thermodynamic) enolate is formed under - + reversible conditions (tBuO K , tBuOH). The less substituted (kinetic) enolate is formed under irreversible conditions (LDA, THF, -78°C). 197 20.3: The Aldol Condensation – An enolate of one carbonyl (nucleophile) reacts with the carbonyl carbon (electrophile) of a second carbonyl compound resulting in the formation of a new C–C bond. Mechanism of the base-catalyzed aldol reaction (Mechanism 20.2, p. 828): O HO H O 2 + – OH H3C C H H3C C C C H H H acetaldehyde 3-hydroxybutanal (β-hydroxyaldehyde) The position of the equilibrium for the aldol reaction is highly dependent on the reaction conditions, substrates, and steric considerations of the aldol product. Low temperature tends to favor the aldol product. 198 99 O H O NaOEt HO H aldol reactions involving α-monosubstituted R C C H R C C EtOH C C H aldehydes are generally favorable H H H H R O H O NaOEt HO H aldol reactions involving α,α-disubstituted R C C H R C C aldehydes are generally unfavorable EtOH C C H R R H R R O H O NaOEt HO R aldol reactions involving ketones are R C C R R C C generally unfavorable EtOH C C R H H H R H The aldol product can undergo acid- or base-catalyzed dehydration to an α,β-unsaturated carbonyl. The dehydration is essentially irreversible. The dehydration is favored at higher temperatures. (mechanism, p. 829) 199 20.4: Mixed and Directed Aldol Reactions – Mixed aldol reaction between two different carbonyl compounds – four possible products (not very useful) HO H O HO H O C C C C O O NaOH, H3CH2CH2CH2C C H H3CH2C C H H2O H3C H H H3CH2CH2C C + H3C C H3CH2CH2C C H C H H H H H HO H O HO H O C C C C H3CH2C C H H3CH2CH2CH2C C H H3C H H H3CH2CH2C Aldehydes with no α-protons can only act as the electrophile O HO H O O O NaOH, HO H C C C + H3C C H2O + C C H C H C H H3CH2C C H H H H3C H H3C H Preferred reactivity HO H O O O NaOH, C C C C H2O H + C CH3 H3C CH 3 H H 200 100 Directed aldol reaction – Discrete generation of an enolate with lithium diisopropyl amide (LDA) under aprotic conditions (THF as solvent) O H3C C HO H O O O Li+ C H LDA, THF, -78°C C C H CH CH C C H H 3 2 2 H3CH2CH2C C H3CH2C C H C H C H H then H2O H CH CH C H H H 3 2 2 O H3CH2CH2C C O O Li+ C H HO H O LDA, THF, -78°C H H C C H3C C H3C C C H C H H3CH2CH2CH2C C H H C H H H H then H2O 3 20.5: Acylation of Enolates: The Claisen Condensation Reaction. Base-promoted condensation of two esters to give a β-keto-ester product O Na+ –OEt, EtOH O O 2 C C C H C OEt + 3 then H3O H3C C OEt H H Ethyl 3-oxobutanoate Ethyl acetate (ethyl acetoacetate) 201 The mechanism of the Claisen condensation (Mechanism 20.3, p. 834) is a base promoted nucleophilic acyl substitution of an ester by an ester enolate and is related to the mechanism of the aldol reaction. 202 101 The Dieckmann Cyclization: An intramolecular Claisen Condensation. The Dieckmann Cyclization works best with 1,6- diesters, to give a 5-membered cyclic β-keto ester product, and 1,7-diesters to give 6-membered cyclic β-keto ester product. O OEt O O EtONa, EtOH OEt EtONa, EtOH O CO Et CO2Et 2 O OEt OEt O Mechanism: same as the Claisen Condensation 203 Mixed Claisen Condensations. Similar restrictions as the mixed aldol condensation. Four possible products: O O O O C C C C O O EtONa, H3CH2CH2CH2C C OEt H3CH2C C OEt EtOH H3C H H H3CH2CH2C C + H3C C H3CH2CH2C C OEt C OEt + H H H H then H3O O O O O C C C C C 5 C3 H3CH2C C OEt H3CH2CH2CH2C C OEt H3C H H H3CH2CH2C Esters with no α-protons can only act as the electrophile O O O EtONa, O EtOH C C C + H3C C OEt C OEt C OEt + H C H H H then H3O 3 Discrete (in situ) generation of an ester enolate with LDA O H3C C O O O O Li+ C OEt LDA, THF, -78°C H H C C H3CH2CH2C C H3CH2CH2C C H3CH2C C OEt C OEt C OEt + H then H2O H CH CH C H H H 3 2 2 O H3CH2CH2C C O O Li+ C OEt O O LDA, THF, -78°C H C C H H C C 3 H3C C H CH CH CH C C OEt C OEt C OEt 3 2 2 2 204 + H C H H H H then H3O 3 102 Acylation of Ketones with Esters.
Details
-
File Typepdf
-
Upload Time-
-
Content LanguagesEnglish
-
Upload UserAnonymous/Not logged-in
-
File Pages18 Page
-
File Size-