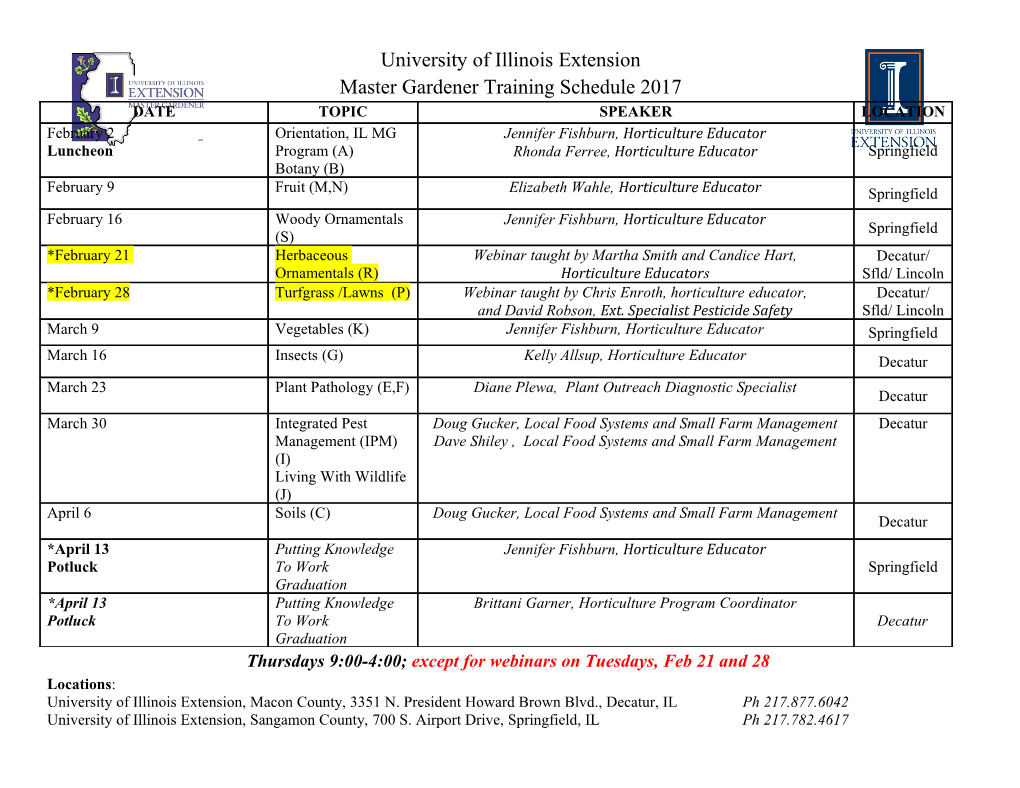
Simulating the density of organic species in the atmosphere of Titan with a coupled ion-neutral photochemical model V. Vuitton, R.V. Yelle, S.J. Klippenstein, S.M. Hörst, P. Lavvas To cite this version: V. Vuitton, R.V. Yelle, S.J. Klippenstein, S.M. Hörst, P. Lavvas. Simulating the density of organic species in the atmosphere of Titan with a coupled ion-neutral photochemical model. Icarus, Elsevier, 2019, 324, pp.120-197. 10.1016/j.icarus.2018.06.013. hal-03038393 HAL Id: hal-03038393 https://hal.archives-ouvertes.fr/hal-03038393 Submitted on 3 Dec 2020 HAL is a multi-disciplinary open access L’archive ouverte pluridisciplinaire HAL, est archive for the deposit and dissemination of sci- destinée au dépôt et à la diffusion de documents entific research documents, whether they are pub- scientifiques de niveau recherche, publiés ou non, lished or not. The documents may come from émanant des établissements d’enseignement et de teaching and research institutions in France or recherche français ou étrangers, des laboratoires abroad, or from public or private research centers. publics ou privés. Simulating the density of organic species in the atmosphere of Titan with a coupled ion-neutral photochemical model V. Vuittona, R. V. Yelleb, S. J. Klippensteinc, S. M. H¨orstd, P. Lavvase aInstitut de Plan´etologie et d'Astrophysique de Grenoble, Univ. Grenoble Alpes, CNRS, Grenoble, 38000, France bLunar and Planetary Laboratory, Univ. of Arizona, Tucson, AZ 85721, USA cChemical Sciences and Engineering Division, Argonne National Lab., Argonne, IL 60439, USA dDepartment of Earth and Planetary Sciences, Johns Hopkins Univ., Baltimore, MD 21218, USA eGroupe de Spectrom´etrieMol´eculaire et Atmosph´erique,Univ. Reims Champagne-Ardenne, CNRS, Reims, 51687, France Abstract We present a one-dimensional coupled ion-neutral photochemical kinetics and diffusion model to study the atmospheric composition of Titan in light of new theoretical kinetics calculations and scientific findings from the Cassini-Huygens mission. The model extends from the surface to the exobase. The atmospheric background, boundary conditions, vertical transport and aerosol opacity are all constrained by the Cassini-Huygens observations. The chemical network in- cludes reactions between hydrocarbons, nitrogen and oxygen bearing species. It takes into account neutrals and both positive and negative ions with masses extending up to about 100 u. We incorporate high-resolution isotopic photoab- sorption and photodissociation cross sections for N2 as well as new photodisso- ciation branching ratios for CH4 and C2H2. Ab initio transition state theory calculations are performed in order to estimate the rate coefficients and products for critical reactions. Main reactions of production and loss for neutrals and ions are quantita- tively assessed and thoroughly discussed. The vertical distributions of neutrals and ions predicted by the model generally reproduce observational data, sug- gesting that for the small species most chemical processes in Titan's atmosphere and ionosphere are adequately described and understood; some differences are highlighted. Notable remaining issues include (i) the total positive ion density (essentially HCNH+) in the upper ionosphere, (ii) the low mass negative ion { { { densities (CN ,C3N /C4H ) in the upper atmosphere, and (iii) the minor oxygen-bearing species (CO2,H2O) density in the stratosphere. Pathways to- wards complex molecules and the impact of aerosols (UV shielding, atomic and molecular hydrogen budget, nitriles heterogeneous chemistry and condensation) are evaluated in the model, along with lifetimes and solar cycle variations. Preprint submitted to Icarus March 16, 2018 Keywords: Titan, atmosphere, Ionospheres, Atmospheres, chemistry, Photochemistry, Organic chemistry 1 1. Introduction 2 Titan is a fascinating world, with its dense (1.5 bars on the surface) and cold 3 (70 - 200 K) atmosphere, and its layers of orange haze that extend to almost 1000 4 km above its surface. As on Earth, the main atmospheric molecule is nitrogen 5 (N2). The second most abundant molecule in Titan's atmosphere is methane 6 (CH4); Titan's surface conditions are near its triple point resulting in a cycle 7 (evaporation, condensation, precipitation) similar to that of water on Earth. 8 Consequently, Titan is the object of the solar system whose superficial structure 9 is the closest to that of the Earth and it has many of the same physical processes 10 as the Earth but they are based on a completely different chemistry. The few 11 percent of methane and the low amount of oxygen (mainly carried by carbon 12 monoxide, CO) make the atmosphere more chemically reducing than present day 13 Earth, where chemistry is based on oxidizing processes linked to the presence 14 of oxygen. The study of Titan's chemistry thus provides the opportunity to 15 determine how the organic nitrogen molecules involved in the chemistry of living 16 organisms (e.g. nitrogen bases, amines ...) can be generated naturally in a 17 planetary environment (H¨orstet al., 2012). 18 Understanding the chemistry that takes place in Titan's atmosphere is an 19 intimidating task because of the multiplicity of chemical and physical processes 20 involved. Chemical processes begin with the dissociation and ionization of N2 21 and CH4 by solar ultraviolet radiation and energetic particles, which provide the 22 H, C, and N atoms necessary for the synthesis of complex organic molecules. The 23 species produced react with the ambient gas to generate a multitude of molecules 24 which will gradually grow to become micron size solid particles, or aerosols. 25 Thus, molecular growth is induced by gas phase reactions which involve radicals 26 as well as positive and negative ions, possibly in excited states and also probably 27 by heterogeneous chemistry on the surface of aerosols. The efficiency and the 28 products of these reactions depend strongly on the physical characteristics of 29 the atmosphere: pressure and temperature. In addition, the distribution of 30 species is affected by molecular diffusion, vertical and horizontal winds, as well 31 as the escape of the lightest compounds and the condensation of the heaviest 32 compounds in the lower stratosphere. A diagram illustrating the atmospheric 33 chemistry of Titan is shown in Figure 1 of H¨orst(2017). 34 The Voyager 1 mission to Saturn in 1980 and subsequent Earth-based obser- 35 vations identified more than a dozen neutral hydrocarbons, nitriles and oxygen- 36 bearing species. From 2004 to 2017, the Cassini spacecraft has observed Titan 37 in situ and by remote sensing and has made exceptional discoveries. In the 38 upper atmosphere, it detected the presence of positively and negatively charged 39 ions with m/z reaching up to thousands of atomic mass units (Waite et al., 40 2007; Coates et al., 2009; Crary et al., 2009; Coates et al., 2010), revealing that 41 Titan has the most compositionally complex ionosphere in the Solar System. It 2 42 became clear that these extremely complex particles are the precursors of the + 43 aerosols known to be present at lower altitude. Also, energetic OHx (x = 0-3) 44 ions have been observed in Saturn's magnetosphere in the vicinity of Titan (Har- 45 tle et al., 2006) and could be at the origin of the oxygen-bearing compounds. 14 15 46 Finally, the N/ N ratios in N2, HCN and CH3CN have been retrieved and are 47 one of the keys to furthering our understanding of the energy sources, primary 48 processes and therefore the evolution of the atmospheric molecular complexity 49 (Vuitton et al., 2014). 50 A large number of chemical models of the atmosphere were created prior 51 to the Cassini mission, with main focus on the ionosphere (Keller et al., 1992; 52 Fox and Yelle, 1997; Keller et al., 1998; Galand et al., 1999), the stratosphere 53 (Yung et al., 1984; Toublanc et al., 1995; Lara et al., 1996), both (Banaszkiewicz 54 et al., 2000; Wilson and Atreya, 2004) or the galactic cosmic rays (GCR) in- 55 duced layer (Molina-Cuberos et al., 1999a,b, 2002). They mostly explained 56 the neutral composition in the stratosphere but their predictions regarding the 57 thermosphere turned out to be incomplete. For example, it was predicted that + + + 58 HCNH ,C2H5 and CH5 would be abundant but many of the ion species de- 59 tected by Cassini were not anticipated. Also, the presence of negative ions was 60 not predicted in the ionosphere. This triggered the re-examination of Titan's 61 upper atmospheric chemistry and put a strong emphasis on ion chemistry. 62 Motivated by the return of the first in situ measurements of Titan's upper 63 atmospheric composition, we developed a 0D ionospheric model to constrain 64 the density of minor neutral constituents (Vuitton et al., 2006b). The model 65 assumed local chemical equilibrium and adopted atmospheric conditions appro- 66 priate for the 1100 km level. The densities of neutral species were fixed at 67 values determined during the outbound portion of the T5 flyby, that was deep 68 into the nightside. The relative abundance of the primary ions was calculated 69 for a mixture of N2 and CH4 irradiated by 100 eV magnetospheric electrons 70 and the magnitude of the ion production was scaled to reproduce the electron 71 density measured by the Langmuir probe. The chemical network included ∼40 72 neutrals, ∼150 ions and ∼1250 reactions. We showed that the most abundant 73 positive ions are closed-shell species because radical cations are not very sta- 74 ble and therefore react quickly. This implies that while odd mass channels are 75 attributed to hydrocarbon ions, peaks at even m/z are the signature of ions con- 76 taining one nitrogen atom. All ion-molecule reactions do not necessarily lead to 77 molecular growth because proton transfer to molecules having a higher proton 78 affinity, typically nitrogen-bearing species, is very efficient.
Details
-
File Typepdf
-
Upload Time-
-
Content LanguagesEnglish
-
Upload UserAnonymous/Not logged-in
-
File Pages249 Page
-
File Size-