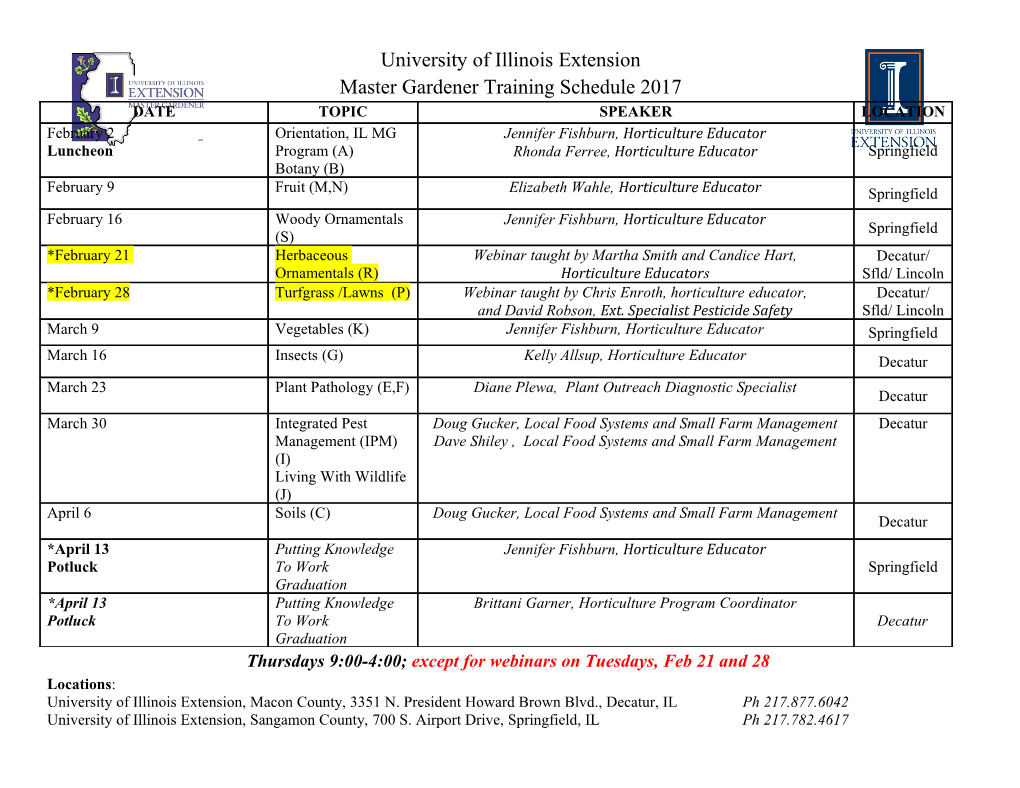
ENGINEERING TITANIUM DIOXIDE-POLY(VINYLIDENE FLUORIDE) AND TITANIUM DIOXIDE-SILICON DIOXIDE CAPACITORS FOR IMPROVED ENERGY STORAGE By Michael Bryant A Thesis Submitted in Partial Fulfillment of the Requirements for the Degree of Master of Science in Applied Physics Northern Arizona University August 2019 Approved: Dr. Randy Dillingham, PhD, Chair Dr. John Gibbs, PhD Dr. Mark Loeffler, PhD ABSTRACT ENGINEERING TITANIUM DIOXIDE-POLY(VINYLIDENE FLUORIDE) AND TITANIUM DIOXIDE-SILICON DIOXIDE CAPACITORS FOR IMPROVED ENERGY STORAGE Michael Bryant Ceramic-polymer capacitors provide a promising ability to improve energy storage due to their averaging effect of high dielectric constant and high dielectric strength materials. Titanium dioxide and poly(vinylidene fluoride) dielectric films were first fabricated in 2011 using a spin coating technique; however, this technique has limited applications within electronics. Within recent years, the dielectric films were fabri- cated using a resistive thermal evaporator, but the quantity of titanium dioxide which could be evaporated and incorporated into the films was found to be limited to 17% of the total dielectric film composition. The purposes of this study are to co-deposit the materials using two separate evaporation techniques to show that doing so is a viable method and to study the electrical properties of a titanium dioxide dominant capacitor. The titanium dioxide and poly(vinylidene fluoride) capacitor is fabricated via physical vapor deposition using thermal resistive evaporation and electron beam gun evaporation. This capacitor is compared to titanium dioxide and silicon dioxide capacitors fabricated using the same methods. The dielectric films are fabricated by co-depositing materials using thermal resistive evaporation and electron beam gun evaporation. The metal electrodes are deposited using sputter coating or electron beam gun evaporation. The dielectric thin films are characterized for their break- down voltage, dielectric strength, capacitance, dielectric constant, thickness, com- position, and surface topography using a scanning electron microscope, an energy ii dispersive X-ray spectrometer, an atomic force microscope, a capacitance measuring RC circuit, and a breakdown voltage tester. Empirical models are created for the titanium dioxide-silicon dioxide capacitors relating the titanium dioxide percentage to the dielectric constant and dielectric strength. The fabricated ceramic-polymer capacitor consisted of 94.10 ± 0.09 % titanium dioxide, and had a breakdown voltage of 19.61 ± 0.01 V, a dielectric strength of 24.15 ± 0.02 MV m−1, a capacitance of 26.123 ± 3.347 nF, a dielectric constant of 33.62 ± 4.45, a thickness of 812.0 ± 0.3 nm, maximum energy storage of 5.02 ± 0.46 µJ, and volume energy density of 0.0868 ± 0.0079 J cm−3. In comparison, the five titanium dioxide and silicon dioxide capacitors had a titanium dioxide composition ranging from 0 % to 100 %, a breakdown voltage ranging from 0.49 ± 0.01 V to 3.25 ± 0.01 V, a dielectric strength ranging from 0.50 ± 0.01 MV m−1 to 18.36 ± 0.06 MV m−1, a capacitance ranging from 54.93 ± 2.43 nF to 150.20 ± 8.19 nF, a dielectric constant ranging from 15.41 ± 0.68 to 87.75 ± 10.79, a thickness ranging from 98.21 ± 0.12 nm to 177.04 ± 0.14 nm, a maximum energy storage ranging from 0.0677 ± 0.00155 µJ to 0.4044 ± 0.0127 µJ, and volume energy density ranging from 0.0103 ± 0.0003 J cm−3 to 0.00970 ± 0.0012 J cm−3. The empirical models suggest that to maximize energy storage through composition, two-fifths of the composition of the dielectric film should be titanium dioxide and three-fifths should be silicon dioxide. When comparing the titanium dioxide and poly(vinylidene fluoride) capacitors to the titanium dioxide and silicon dioxide capacitors, the capacitor with poly(vinylidene fluoride) exhibited superior energy storage and energy density properties. iii ACKNOWLEDGMENTS I would like to thank the following people for their help and support: Dr. Randy Dillingham, committee chair Dr. John Gibbs, committee member Dr. Mark Loeffler, committee member Dr. William "Buzz" Delinger, electronics advisor Dr. Dylan Nicholls, Nanomaterials Laboratory postdoctoral researcher Joel Johnson, Nanomaterials Laboratory graduate researcher Taylor Thomas, Nanomaterials Laboratory graduate researcher Aubrey Funke, Imaging Histology Core Facility Assistant Director Miles Knight, undergraduate research assistant Cameron Richards, undergraduate research assistant My family and friends. iv Contents 1 Introduction 1 2 Background 5 2.1 Capacitors . .5 2.2 Thin Film Deposition . .7 2.2.1 Physical Vapor Deposition . .8 2.3 Materials . 11 2.3.1 Ceramics . 12 2.3.2 Polymers . 13 2.3.3 Composites . 13 3 Methods 16 3.1 Purpose . 16 3.2 Deposition Equipment . 17 3.3 Capacitor Fabrication Process Overview . 20 3.4 Material Preparation . 20 3.5 Film Deposition Procedure . 21 3.5.1 Electrodes . 21 3.5.2 Dielectric Film . 22 3.6 Film Analysis . 24 3.6.1 Structure . 25 v 3.6.2 Composition . 30 3.6.3 Electrical Properties . 32 4 Results and Discussion 34 4.1 Overview . 34 4.1.1 Titanium Dioxide-Poly(vinylidene Fluoride) Capacitor . 34 4.1.2 Titanium Dioxide-Silicon Dioxide Capacitors . 35 4.2 Titanium Dioxide-Poly(vinylidene Fluoride) Films and Capacitor . 36 4.2.1 Deposition Current, Composition, and Surface Features of the Films . 37 4.2.2 Composition, Structure, and Electrical Properties of the Ca- pacitor . 45 4.2.3 Prediction of Composition to Maximize Energy Stored using Literature Values . 52 4.3 Titanium Dioxide-Silicon Dioxide Film and Capacitors . 55 4.3.1 Surface Features of the Film . 56 4.3.2 Composition, Structure, and Electrical Properties of the Ca- pacitors . 57 5 Conclusions and Future Work 68 A Designing, Building, and Automating a High-Vacuum Physical Va- por Deposition System 70 B LabVIEW: Breakdown Voltage Measuring Circuit 75 C Additional Results Data 80 C.1 Titanium Dioxide-Poly(vinylidene Fluoride) Films . 80 C.2 Titanium Dioxide-Poly(vinylidene Fluoride) Capacitor . 83 C.3 Titanium Dioxide-Silicon Dioxide Capacitors . 84 vi D Statistical Methods 94 E MATLAB: Results Calculations 96 Bibliography 111 vii List of Figures 2.1 A model of a parallel-plate capacitor. .6 2.2 A diagram of an electron gun bombarding material with electrons, and the resulting vaporization and byproducts of the process. 10 2.3 A diagram of a glow discharge sputter coater with argon ions bombard- ing a target material which causing the material to gain momentum and coat a substrate. 11 2.4 A diagram illustrating the phase transformations and corresponding temperatures for titanium dioxide. 13 3.1 A model and diagram of the Torr International, Inc. deposition system. 19 3.2 A diagram of the products of the capacitor fabrication process. 20 3.3 A model of the Zeiss Supra 40VP scanning electron microscope. 27 3.4 The spectrum produced by the energy dispersive X-ray spectrometer of elements in the titanium dioxide-poly(vinylidene fluoride) capacitor. 31 4.1 A graph comparing the composition to the dielectric constant for the five titanium dioxide-silicon dioxide capacitors. 35 4.2 A graph comparing the composition to the dielectric strength for the five titanium dioxide-silicon dioxide capacitors. 36 4.3 The phase image produced by the atomic force microscope of Film 7. 40 4.4 The phase image produced by the atomic force microscope of Film 8. 41 viii 4.5 The phase image produced by the atomic force microscope of Film 9. 41 4.6 The phase image produced by the atomic force microscope of Film 10. 42 4.7 The phase image produced by the atomic force microscope of the poly(vinylidene fluoride) dominant side of the titanium dioxide-poly(vinylidene fluoride) film. 43 4.8 The phase image produced by the atomic force microscope phase image of the middle of the titanium dioxide-poly(vinylidene fluoride) film. 43 4.9 The phase image produced by the atomic force microscope phase im- age of the titanium dioxide dominant side of the titanium dioxide- poly(vinylidene fluoride) film. 44 4.10 The carbon elemental map produced by the scanning electron mi- croscope and energy dispersive X-ray spectrometer for the titanium dioxide-poly(vinylidene fluoride) capacitor. 45 4.11 The fluorine elemental map produced by the scanning electron mi- croscope and energy dispersive X-ray spectrometer for the titanium dioxide-poly(vinylidene fluoride) capacitor. 46 4.12 The titanium elemental map produced by the scanning electron mi- croscope and energy dispersive X-ray spectrometer for the titanium dioxide-poly(vinylidene fluoride) capacitor. 46 4.13 The oxygen elemental map produced by the scanning electron mi- croscope and energy dispersive X-ray spectrometer for the titanium dioxide-poly(vinylidene fluoride) capacitor. 47 4.14 The carbon and fluorine elemental maps produced by the scanning electron microscope and energy dispersive X-ray spectrometer overlaid on top of scanning electron microscope image for the titanium dioxide- poly(vinylidene fluoride) capacitor. 48 ix 4.15 The titanium and oxygen elemental maps produced by the scanning electron microscope and energy dispersive X-ray spectrometer overlaid on top of scanning electron microscope image for the titanium dioxide- poly(vinylidene fluoride) capacitor. 49 4.16 The cross-sectional image produced by the scanning electron micro- scope of the titanium dioxide-poly(vinylidene fluoride) capacitor with thickness measurements. 50 4.17 A graph comparing the composition to the literature values for the dielectric constant for titanium dioxide-poly(vinylidene fluoride) ca- pacitors. 53 4.18 A graph comparing the composition to the literature values for the di- electric strength for titanium dioxide-poly(vinylidene fluoride) capacitors. 54 4.19 The plot of the product of the dielectric constant and dielectric strength squared as a function of titanium dioxide concentration for titanium dioxide-poly(vinylidene fluoride) capacitors.
Details
-
File Typepdf
-
Upload Time-
-
Content LanguagesEnglish
-
Upload UserAnonymous/Not logged-in
-
File Pages126 Page
-
File Size-