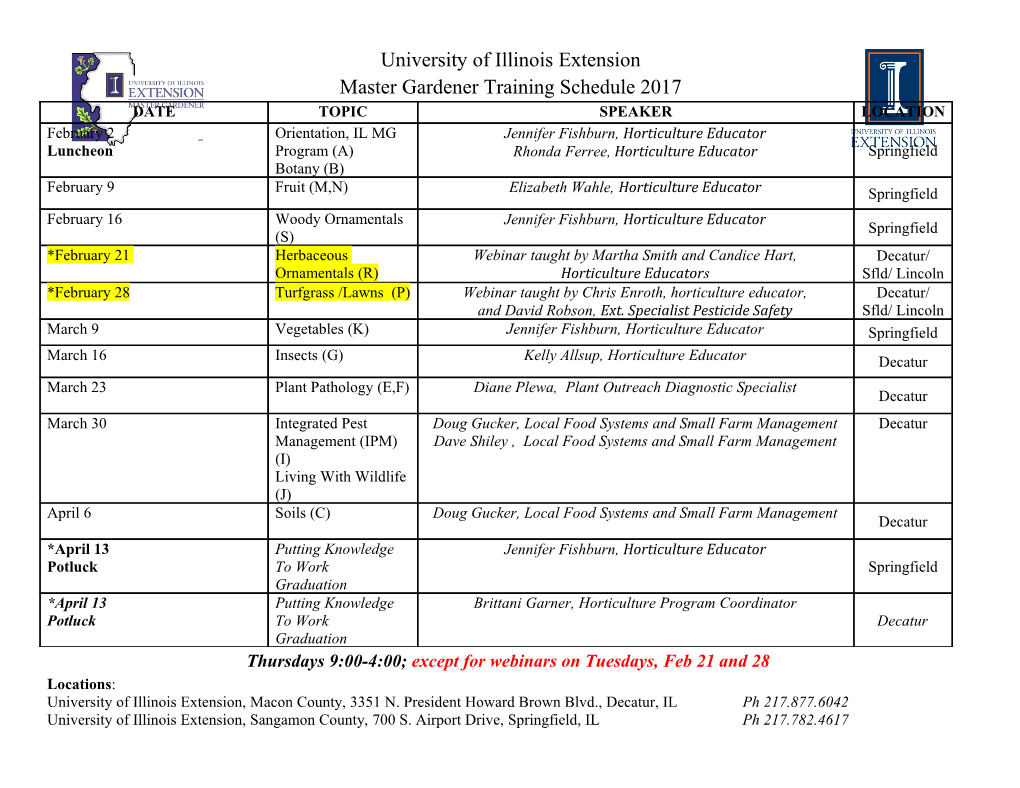
cells Review The Immuno-Modulatory Effects of Inhibitor of Apoptosis Protein Antagonists in Cancer Immunotherapy Jessica Michie 1,2, Conor J. Kearney 1,2, Edwin D. Hawkins 1,3, John Silke 3,4 and Jane Oliaro 1,2,5,* 1 Peter MacCallum Cancer Centre, Melbourne, VI 3000, Australia; [email protected] (J.M.); [email protected] (C.J.K.); [email protected] (E.D.H.) 2 Sir Peter MacCallum Department of Oncology, The University of Melbourne, VI 3010, Australia 3 The Walter and Eliza Hall Institute of Medical Research, VI 3010, Australia; [email protected] 4 Department of Medical Biology, University of Melbourne, Parkville, VI 3010, Australia 5 Department of Immunology, Monash University, Melbourne, VI 3004, Australia * Correspondence: [email protected]; Tel.: +61-3-8559-7094 Received: 29 November 2019; Accepted: 11 January 2020; Published: 14 January 2020 Abstract: One of the hallmarks of cancer cells is their ability to evade cell death via apoptosis. The inhibitor of apoptosis proteins (IAPs) are a family of proteins that act to promote cell survival. For this reason, upregulation of IAPs is associated with a number of cancer types as a mechanism of resistance to cell death and chemotherapy. As such, IAPs are considered a promising therapeutic target for cancer treatment, based on the role of IAPs in resistance to apoptosis, tumour progression and poor patient prognosis. The mitochondrial protein smac (second mitochondrial activator of caspases), is an endogenous inhibitor of IAPs, and several small molecule mimetics of smac (smac-mimetics) have been developed in order to antagonise IAPs in cancer cells and restore sensitivity to apoptotic stimuli. However, recent studies have revealed that smac-mimetics have broader effects than was first attributed. It is now understood that they are key regulators of innate immune signalling and have wide reaching immuno-modulatory properties. As such, they are ideal candidates for immunotherapy combinations. Pre-clinically, successful combination therapies incorporating smac-mimetics and oncolytic viruses, as with chimeric antigen receptor (CAR) T cell therapy, have been reported, and clinical trials incorporating smac-mimetics and immune checkpoint blockade are ongoing. Here, the potential of IAP antagonism to enhance immunotherapy strategies for the treatment of cancer will be discussed. Keywords: smac-mimetics; TNF; cancer immunotherapy; checkpoint blockade; CAR T cells 1. Inhibitor of Apoptosis Proteins The capacity to evade apoptosis, a form of physiological cell death that relies on the activation of a family of cysteine proteases known as caspases [1], is a common trait of malignantly transformed cells [2]. During apoptotic cell death, endogenous second mitochondrial activator of caspases/Direct IAP-Binding Protein With Low PI (smac/DIABLO), is released from the mitochondrial inter-membrane space where it binds to, and inhibits, the three major inhibitor of apoptosis proteins; cellular IAP 1 (cIAP1, BIRC2) and 2 (cIAP2, BIRC3) and X-linked IAP (XIAP, BIRC4)[3,4]. The inhibitor of apoptosis (IAP) proteins are a family of endogenous proteins that function as key regulators of caspase activity, and are defined by the presence of at least one Baculoviral IAP Repeat (BIR) domain. These approximately 70-residue zinc-binding domains enable their interaction with, and suppression of, caspases, and therefore facilitate the inhibition of apoptosis [5]. Only XIAP is a potent direct inhibitor of caspases, Cells 2020, 9, 207; doi:10.3390/cells9010207 www.mdpi.com/journal/cells Cells 2020, 9, 207 2 of 16 however, the physiological significance of this activity is unclear, because cells from patients with XIAP mutations [6] and murine XIAP knockout mice, are not more sensitive to apoptosis than wild type cells [7]. Importantly, IAPs also contain a RING finger E3 ligase domain at the C-terminus [8,9], enabling these proteins to participate in diverse cellular processes, including signal transduction events that promote inflammation, cell cycle progression and migration. Notably, IAPs are critical regulators of both canonical and alternative (non-canonical) nuclear factor kappa light-chain enhancer of activated B cells (NF-κB) signalling, downstream of various members of the Tumour Necrosis Factor Receptors Superfamily (TNFRSF). 1.1. Inhibitor of Apoptosis Proteins in NF-κB Signalling IAPs are required for the activation of the canonical NF-κB pathway downstream of several receptors [10,11]. One of the best studied is downstream of TNF Receptor 1 (TNFR1) (Figure1). In this pathway, TNFR1 ligation by TNF results in the formation of a complex comprising RIPK1, TRADD, and TRAF2 (Complex I), where TRAF2 is the primary factor required for the recruitment of IAPs [12–14]. IAPs ubiquitylate several components within this complex, although the best studied is RIPK1 [15–18]. The downstream signalling pathway consists of the trimeric canonical IκB kinase (IKK) complex, composed of IKKα and IKKβ subunits, as well as the regulatory subunit IKKγ (also known as NF-κB essential modulator (NEMO)). IAP-mediated ubiquitylation of Complex I mediates the recruitment of the linear ubiquitin chain assembly complex (LUBAC) [19], which is comprised of HOIL-1L, HOIP and Sharpin [20]. LUBAC generates M1 linked ubiquitin chains on Complex I components such as RIPK1 and IKKγ [21], which stabilizes Complex I and allows full activation of the IKK complex (consisting of IKK1, IKK2 and IKKγ/NEMO) and a TAK1 containing complex. IKK2 phosphorylates IκBα, resulting in its proteasomal degradation and the release of the p50 and p65/RelA NF-κB heterodimer, which allows their translocation to the nucleus [22,23], while TAK1 activation leads to activation of the MAPK pathway. This results in the induction of pro-survival and inflammatory transcriptional programs [24]. Figure 1. The Inhibitor of Apoptosis Proteins (IAPs) are critical regulators of both canonical and non-canonical NF-κB signalling. During canonical NF-κB signalling, the ubiquitylation of Complex I Cells 2020, 9, 207 3 of 16 components by cIAPs results in the nuclear translocation and activation of pro-survival canonical NF-κB and limits the formation of pro-apoptotic Complex II. cIAPs also target NIK for proteasomal degradation preventing the activation of non-canonical NF-κB. Loss of IAPs results in the formation of Complex II and activates caspase-mediated apoptosis, and results in the accumulation of NIK, which causes downstream non-canonical NF-κB activation. IAP-mediated ubiquitylation of RIPK1 in Complex I also limits RIPK1 association with FADD and caspase 8 to form the ripoptosome (Complex II) [25]. Together MAPK, IKK activation and IAP ubiquitylation therefore suppress TNF induced apoptosis. As a result, antagonism, or the absence of, IAPs results in signalling through TNFR1 that activates caspase-mediated apoptosis, rather than the induction of NF-κB pro-survival signalling [26–28]. IAPs also inhibit cell death induced by the TNFSF death ligands, FasL and TRAIL, as well as chemotherapy agents [28–31]. In these cases, it is likely that preventing RIPK1 from generating a FADD caspase-8 activating complex is the primary mechanism of inhibition, while NF-κB activation plays a less significant role. The cellular IAPs (cIAPs) also have a critical role in the regulation of NF-κB-inducing kinase (NIK) through K-48 ubiquitylation; a process that leads to its continuous degradation and therefore low-level expression [26,27]. Therefore, the loss or inhibition of IAPs leads to NIK stabilization and accumulation, phosphorylation and activation of IKKα, phosphorylation and processing of p100 (NFκB2) and nuclear translocation of the p52 and RelB NF-κB heterodimer; resulting in activation of the so-called non-canonical NF-κB pathway. 1.2. IAPs and Smac-Mimetics in Cancer IAPs are amplified in approximately 3% of cancers [32], and several IAPs can act as proto-oncogenes as a result of genetic alterations in various cancer types [33]. As IAPs promote cell survival in response to TNFSF ligands and chemotherapy, their upregulation renders cancer cells resistant to the cytotoxic effects of these agents, and is associated with failure of treatment and poor prognosis in multiple cancer types [24,25,34]. For example, XIAP expression has been implicated in cisplatin resistance in ovarian cancer [35], correlated with disease severity in AML [36], and is a prognostic indicator in renal cell carcinoma [37]. Following the discovery of a potent antagonist of XIAP [38], small molecule mimetics of smac/DIABLO (smac-mimetics; SMs) were developed to overcome the survival benefits of IAP upregulation in cancer cells, through their antagonism of IAP activity and promotion of tumour cell death [39–42]. SMs promote IAP degradation by binding to the BIR3 domain of cIAPs, which allows the RING to dimerize, and in turn, activate the E3 ubiquitin ligase function of the cIAPs [26,27,43]. As they are not bound to a natural substrate such as NIK, this results in their own ubiquitylation and degradation [44,45]. Although SMs also bind to the BIR3 of XIAP, very few of them promote degradation of XIAP, although they nevertheless can antagonise normal XIAP function [11,46,47]. A number of SMs have been developed over the past decade with differing chemistries, including monovalent and bivalent varieties, which both mimic the N-terminus of SMAC and subsequently promote RIPK1-caspase8 complex formation and apoptosis. However, bivalent SMs are typically more cytotoxic than the structurally related monovalent IAP antagonists. Biochemical analyses of various monovalent and bivalent SMs have revealed that bivalency promotes cIAP1 degradation more effectively than monovalent IAP antagonists, and monovalent SMs are also less effective at depleting TRAF2-associated cIAP1, and thus inhibiting canonical NF-κB signalling [48]. SMs not only degrade IAPs to promote tumor cell death, but also induce the production of TNF in a subset of tumor cells, which can act in an autocrine or paracrine manner to cause tumor cell death [24,26,27,49,50]. In this manner, single agent efficacy of SMs has been reported, particularly for the treatment of certain leukaemias [51–53].
Details
-
File Typepdf
-
Upload Time-
-
Content LanguagesEnglish
-
Upload UserAnonymous/Not logged-in
-
File Pages16 Page
-
File Size-