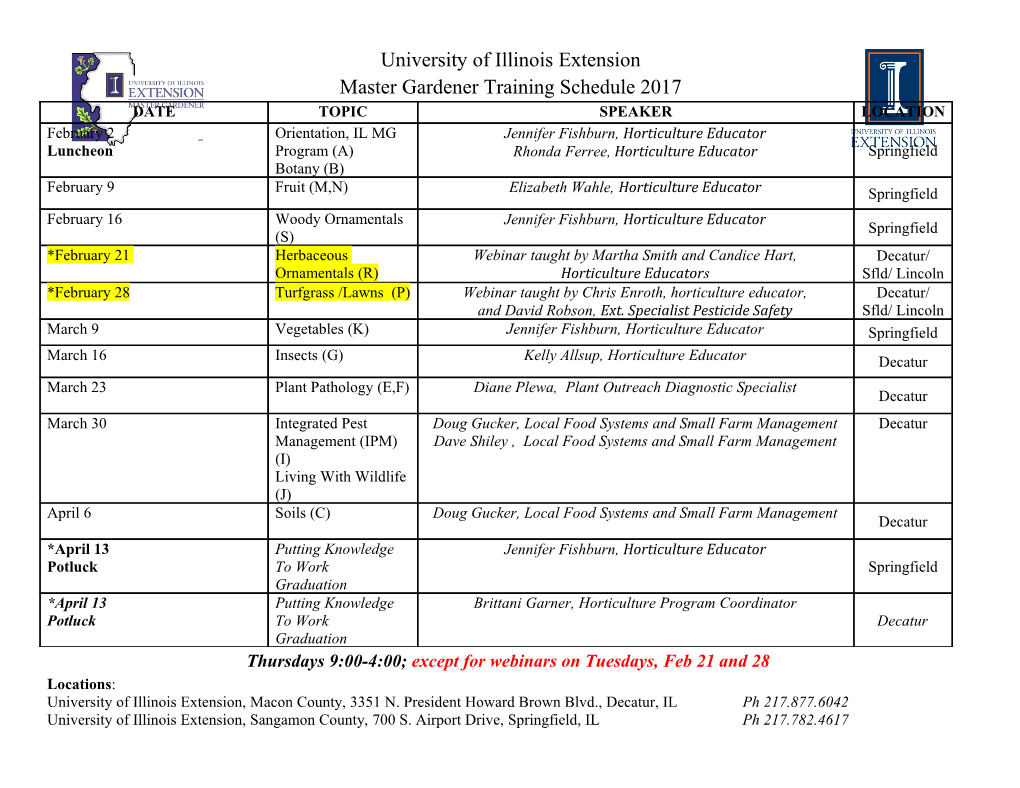
MIAMI UNIVERSITY The Graduate School Certificate for Approving the Dissertation We hereby approve the Dissertation of James D. Garrity Candidate for the Degree: Doctor of Philosophy _______________________________________________ Director Dr. Michael Crowder _______________________________________________ Reader Dr. Gilbert Gordon _______________________________________________ Reader Dr. Richard Taylor _______________________________________________ Reader Dr. Hongcai Zhou _______________________________________________ Graduate School Representative Dr. Joyce Fernandes ABSTRACT CHARACTERIZATION OF L1, THE METALLO-β-LACTAMASE FROM Stenotrophomonas maltophilia by James D. Garrity Zinc-containing metallo-β-lactamases are an emerging class of enzymes that render bacteria resistant to β-lactam-containing antibiotics. In an effort to better understand the function of the metallo-β-lactamase L1 from Stenotrophomonas maltoplia, spectroscopic and mechanistic studies were performed. Mutagenesis and biochemical assays of nine L1 active site residues, predicted from computational studies to be important for tight substrate binding to the enzyme, revealed that none of the residues play a significant role in the binding of substrate to L1. Investigation of a metal-binding aspartic acid showed that, in addition to ligating a Zn(II), this residue also plays an important role in catalytic cycle of the enzyme by properly orienting a water molecule that takes part in a rate-limiting protonation event. Fluorescence studies revealed that the rate of motion of a flexible loop of amino acids that extends over the active site of L1 correlates to the formation of a non-rate-limiting intermediate in the catalytic cycle of L1. Rapid-scanning and rapid-freeze quench EPR spectrophotometry studies provided the first direct evidence that the reaction intermediate of L1 is metal bound. This dissertation offers data, which, along with previous results on L1 and other metallo-β-lactamases, can be integrated and used to guide further rational inhibitor design efforts. CHARACTERIZARTION OF L1, THE METALLO-β-LACTAMASE FROM Stenotrophomonas maltophilia A DISSERTATION Submitted to the Faculty of Miami University in partial fulfillment of the requirements for the degree of Doctor of Philosophy Department of Chemistry and Biochemistry by James D. Garrity Miami University Oxford, Ohio 2004 Dissertation Director: Dr. Michael Crowder TABLE OF CONTENTS Chapter 1 Introduction 1 1.1 Antibiotics 2 1.2 β-lactam Containing Antibiotics 2 1.3 Antibiotic Resistance 8 1.4 Mechanism of Resistance 9 1.5 β-Lactamases 10 1.5.1 Serine-β-Lactamases 10 1.5.2 Metallo-β-Lactamases 12 1.5.2.1 Zinc Metallo-Hydrolase Family of Proteins 16 1.6 Stenotrophomonas maltophilia 18 1.7 Antibiotic Resistance in Stenotrophomonas maltophilia 20 1.8 Introduction to Dissertation 23 1.8.1 Rational Drug Design 23 1.8.2 Sections of the Dissertation 24 1.9 References 25 Chapter 2 Probing Substrate Binding to Metallo-β-Lactamase L1 34 from Stenotrophomonas maltophilia by Using Site-Directed Mutagenesis 2.1 Summary 36 2.2 Introduction 37 2.3 Materials and Methods 41 ii 2.4 Results 45 2.4.1 Wild Type L1 45 2.4.2 Ser224 Mutants 52 2.4.3 Asn233 Mutants 56 2.4.4 Tyr228 Mutants 57 2.4.5 Ile164 and Phe158 Mutants 60 2.5 Discussion 62 2.6 Conclusion 68 2.7 Acknowledgements 70 2.8 References 71 Chapter 3 Metal binding Asp120 in metallo-β-lacatamse L1 from 77 Stenotrophomonas maltophilia plays a crucial role in catalysis 3.1 Summary 79 3.2 Introduction 80 3.3 Eperimental Procedures 85 3.4 Results 90 3.5 Discussion 99 3.6 Acknowledgements 105 3.7 References 106 iii Chapter 4 Probing the dynamics of a mobile loop above the active 112 site of L1, a metallo-β-lactamase from Stenotrophomonas maltophilia, via site-directed mutagenesis and stopped-flow fluorescence spectroscopy. 4.1 Summary 114 4.2 Intoduction 115 4.3 Experimental Procedures 119 4.4 Results 123 4.5 Discussion 134 4.6 Acknowledgements 141 4.7 References 142 Chapter 5 First Direct Evidence that the Reaction Intermediate of 147 Metallo-β-Lactamase L1 is Metal Bound 5.1 Summary 149 5.2 Introduction 150 5.3 Experimental Procedures 154 5.4 Results 157 5.5 Discussion 167 5.6 References 175 iv Chaper 6 Conclusions 180 6.1 The Growing Problem of Antibiotic Resistance 180 6.2 Direct Inhibition of Metallo-β-lactamases 182 6.3 Indirect Inhibition of Metallo-β-lactamases 184 6.4 References 186 v LIST OF FIGURES 1-1: There are multiple sites for antibiotic attack in a bacterial cell. 3 1-2: Core structures of common β-lactam containing antibiotics. 4 1-3: Crosslinking of building blocks of the peptidoglycan layer 6 1-4: Cell wall biosynthesis showing the mode of inhibition for β-lactam 7 antibiotics. 1-5: Hydrolysis of Imipenem. 11 1-6: Amino acid comparison of conserved segments of the zinc metallo- 17 hydrolase family of enymes. 2-1: Active site residues that were mutated in this study. 40 2-2: CD spectra of wild-type L1 and L1 mutants. 47 2-3: Intermediate formation by wild-type L1 and L1 mutants. 55 2-4 A: Stopped-flow fluorescence of wild-type L1 with nitrocefin. 59 2-4 B: Plot of observed rate constant versus concentration of nitrocefin. 59 3-1: Pictorial representation of the active site of wild-type L1 rendered 84 using Chem Draw Ultra v. 5.0 (top) and Rasmol 2.6 (bottom). 3-2: Formation of ring-opened, nitrogen anion intermediate by 94 wild-type L1 and L1 mutants. 3-3: pH dependence plots of wild-type L1 with (A) nitrocefin, 96 ∆ kcat, ◊ kcat/Km, and (B) penicillin G, Ο kcat, kcat/Km. 3-4: pH dependence plots of L1 mutants with nitrocefin. 97 3-5: Proton inventory plots of wild-type L1 and L1 mutants with nitrocefin. 98 3-6: Proposed role of Asp120 in L1. 103 4-1: Structure of L1 monomer showing the positions of key residues 118 involved in this study. vi 4-2: Fluorescence scans of wild-type L1 and L1 mutants. 127 4-3: Fluorescence titrations of apo wild-type L1 and L1 mutants. 129 4-4: Stopped-flow fluorescence spectra of wild-type L1 and L1 mutants 130 with nitrocefin. 4-5: Stopped-flow fluorescence spectra of W39F, D160W with nitrocefin. 131 4-6: Fluorescence scans of W39F, D160W, free enzyme and 1:1 solutions 132 with nitrocefin and meropenem. 4-7: Single wavelength versus time plots of wild-type L1, W39F, and 133 W39F, D160W with nitrocefin. 5-1: Proposed metal binding site of metallo-β-lactamase L1 when bound 152 to reaction intermediate. 5-2: Rapid-scanning electronic absorption spectra of the reaction of 158 100 µM wild-type L1 with 100 µM nitrocefin at 2 oC. 5-3: Rapid-scanning electronic absorption spectra of the reaction of 158 100 µM Co(II)-substituted L1 with 100 µM nitrocefin at 2 oC. 5-4: EPR spectra and simulations of Co(II)-substituted L1 with 163 nitrocefin as the substrate. 5-5: EPR spectra and simulations of Co(II)-substituted L1 with 164 cephalothin as the substrate. 5-6: EPR spectra and simulations of Co(II)-substituted L1 with 165 meropenem as the substrate. 5-7: EPR spectra and simulations of Co(II)-substituted L1 with 166 penicillin G as the substrate. vii LIST OF TABLES 1-1: Classification schemes for β-lactamases. 13 1-2: Bush group 3 metallo-β-lactamase sub-grouping scheme. 15 2-1: Oligonucleotides used in preparation of L1 mutants. 43 2-2: Metal Content of Wild-type L1 and L1 mutants. 48 2-3: Steady-state kinetic constants for hydrolysis of cephalosporins by 50 wild-type L1 and L1 mutants. 2-4: Steady-state kinetic constants for hydrolysis of penicillins by 51 wild-type L1 and L1 mutants. 2-5: Steady-state kinetic constants for hydrolysis of carbapenems 52 by wild-type L1 and L1 mutants. 2-6: Km and KS values for Wild-type L1 and mutants with nitrocefin 60 as substrate. 3-1: Metal analysis, and Ks and kH/kD values with nitrocefin of 90 wild-type L1 and L1 mutants. 3-2: Steady-state kinetics constants for wild-type L1 and L1 mutants 92 with (2a) carbapenems, (2b) cephalosporins, and (2c) penicillins. 4-1: Analysis of CD Spectra using CDSSTR with a 43 protein reference 124 set optimized for 190-240 nm. 4-2: Steady state kinetics constants for wild-type L1 and L1 mutants. 125 4-3: Summary of fit constants for stopped-flow rapid-scanning 133 and fluorescence data of enzymes with nitrocefin at 2 °C, using IgorPro v 4.0 graphing program. viii Acknowledgements I would like to sincerely thank my advisor, Dr. Michael Crowder. Leading by example, he encouraged positive interaction in the lab, and promoted the sharing of knowledge and technical expertise among his students. His guidance, support, and friendship were invaluable to the completion of this effort. I would also like to thank my committee, Drs. Gordon, Lorigan, Taylor and Fernandes, for their support of my studies at Miami University, and Dr. Hongcai Zhou for substituting on my committee for my final defense. Additionallly, I would like to thank Gerg Patton, Lissa Herron, and Jim Pauff for their efforts in this endeavor. Finally, I would like to dedicate this dissertation to Lisa and my parents. To Lisa, for her day-to-day unconditional love, support, and encouragement - this made the difficult times bearable, and the good times even better. And to my parents, who, by example, have taught me what is important in life; and always given me the love, encouragement, and support necessary to succeed. ix Chapter 1 Introduction Prior to Pasteur’s discovery of a connection between bacteria and disease, a few people had worked diligently in an attempt to make life better by minimizing the effects of microorganisms.
Details
-
File Typepdf
-
Upload Time-
-
Content LanguagesEnglish
-
Upload UserAnonymous/Not logged-in
-
File Pages198 Page
-
File Size-