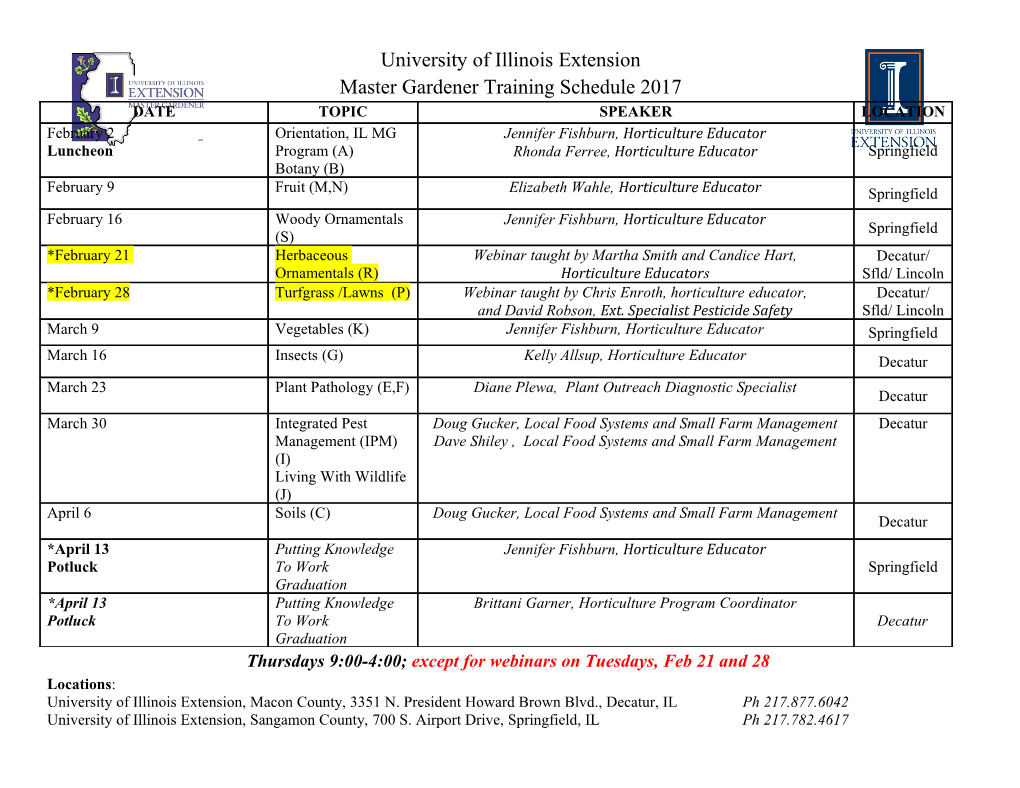
SnapShot: ER-Associated Protein Degradation Pathways Shinichi Kawaguchi and Davis T.W. Ng Temasek Life Sciences Laboratory and Department of Biological Sciences, National University of Singapore, Singapore 117604 1230 Cell 129, June 15, 2007 ©2007 Elsevier Inc. DOI 10.1016/j.cell.2007.06.005 See online version for legend and references. SnapShot: ER-Associated Protein Degradation Pathways Shinichi Kawaguchi and Davis T.W. Ng Temasek Life Sciences Laboratory and Department of Biological Sciences, National University of Singapore, Singapore 117604 Arrows specify the routes of individual pathways. All pathways culminate in substrate degradation by the 26S proteasome. (Left) ERAD Pathways in Budding Yeast (A) Newly synthesized secretory and membrane proteins enter the ER through the Sec61 protein-conducting channel complex unfolded. Hsp70-related molecular chaperones (Kar2p) bind to nascent polypeptides in the ER lumen and to the cytosolic domains of membrane proteins (Hsp70, B). These factors assist in substrate folding and also assist in their disposal if they fail to fold. Mannose residues on misfolded glycoproteins are trimmed by the ER mannosidase Mns1p (E). Mannose trimming facilitates the recognition of misfolded glycoproteins by luminally oriented lectin factors Htm1p and Yos9p. (C, D, and F) At least two ER membrane-localized E3 ubiquitin ligases organize protein complexes that receive and process misfolded proteins. These complexes define three pathways that recognize lesions in the cytosolic (ERAD-C), transmembrane (ERAD-M), and luminal (ERAD-L) domains of substrates. Both ERAD-M and ERAD-L use the Hrd1 ubiquitin ligase but the luminal factor Yos9p is dispensable for ERAD-M. A Hrd1 complex lacking Yos9p has been observed suggesting dedicated complexes for all three pathways. As ubiquitina- tion and degradation occurs in the cytosol, luminal substrates must be retrotranslocated. The identity of the conduit remains unresolved in the ubiquitin-dependent pathways, but the Sec61 complex and Der1 family members have been implicated. (G) In the yeast ubiquitin-independent pathway, misfolded proα-factor in yeast exits via Sec61. Cdc48p/p97 and its cofactors Npl4p and Ufd1p provide the driving force for the extraction of ubiquitinated luminal and membrane substrates from the ER membrane. In association with the Cdc48p/p97 complex, the E4 enzyme Ufd2p remodels ubiquitin chains. The polyubiquitinated substrate is transferred to the Rad23p or Dsk2p complexes assisted through an association with Ufd2p (H). N-linked glycans are removed by Png1p. Finally, the substrate is targeted by this complex to the proteasome where it is degraded (I). (Right) ERAD Pathways in Mammals (a) Similar to yeast, newly synthesized secretory and membrane proteins enter the ER through the Sec61 protein and Hsp70-related molecular chaperones (Bip) bind to nascent polypeptides in the ER lumen. (b) Under conditions of stress, mammalian cells can detect nascent polypeptides delayed in protein translocation. P58IPK recruits cytosolic Hsp70 to attenuate translocation and target the client protein to the proteasome (cotranslocational degradation) as a preemptive measure to limit ER overload. Also, as in yeast mannose residue(s) on misfolded glycoproteins are trimmed by the ER mannosidase I (f), and/or EDEM, EDEM2, and EDEM3, which facilitates their recognition by luminally oriented lectin-like factors EDEM1/2/3. (c, d, e, and g) Mammalian cells use at least four E3 ubiquitin ligases in ERAD (CHIP, RMA1, gp78, and Hrd1). One of these, Hrd1, and a fifth named TEB4 (whose function in ERAD remains to be established) are homologous to the yeast Hrd1p and Doa10p, suggesting evolutionary conservation of the basic ERAD machinery. Similar to the yeast paradigm, each E3 handles a different set of substrates. Depicted with the E3s are known associated factors (SEL1 is the mammalian ortholog of yeast Hrd3p; ERASIN is the mammalian homolog of yeast Ubx2; the Yos9p homolog OS-9 has not yet been shown to be part of the mammalian Hrd1 complex). The mammalian E3 complexes likely contain additional factors that are yet to be identified. Substrate retrotransloca- tion appears to occur via Derlin family members, but the Sec61 complex has not been ruled out for all substrates. The polyubiquitinated substrate is transferred to the Rad23p complexe assisted through an association with the E4 enzyme Ufd2p, which remodels ubiquitin chains (h). N-linked glycans are removed by Png1p and the polyubiquitin chain can be shortened by Atx3. Finally, the substrate is targeted by this complex to the proteasome and degraded (i). Abbreviations Atx3, ataxin-3; CFTR, Cystic fibrosis transmembrane conductance regulator; CHIP, the C terminus of HSP70-interacting protein; calreticulin; ER, endoplasmic reticu- lum; ERAD, ER-associated degradation; EDEM, ER degradation enhancing α-mannosidase-like protein; OST, oligosaccharyltransferase. REFERENCES Hassink, G., Kikkert, M., van Voorden, S., Lee, S.J., Spaapen, R., van Laar, T., Coleman, C.S., Bartee, E., Fruh, K., Chau, V., and Wiertz, E. (2005). TEB4 is a C4HC3 RING finger-containing ubiquitin ligase of the endoplasmic reticulum. Biochem. J.388 , 647–655. Ismail, N., and Ng, D.T. (2006). Have you HRD? Understanding ERAD is DOAble! Cell 126, 237–239. Kim, I., Ahn, J., Liu, C., Tanabe, K., Apodaca, J., Suzuki, T., and Rao, H. (2006). The Png1-Rad23 complex regulates glycoprotein turnover. J. Cell Biol. 172, 211–219. McClellan, A.J., Tam, S., Kaganovich, D., and Frydman, J. (2005). Protein quality control: chaperones culling corrupt conformations. Nat. Cell Biol. 7, 736–741. Meusser, B., Hirsch, C., Jarosch, E., and Sommer, T. (2005). ERAD: the long road to destruction. Nat. Cell Biol. 7, 766–772. Moremen, K.W., and Molinari, M. (2006). N-linked glycan recognition and processing: the molecular basis of endoplasmic reticulum quality control. Curr. Opin. Struct. Biol. 16, 592–599. Oda, Y., Okada, T., Yoshida, H., Kaufman, R.J., Nagata, K., and Mori, K. (2006). Derlin-2 and Derlin-3 are regulated by the mammalian unfolded protein response and are required for ER-associated degradation. J. Cell Biol. 172, 383–393. Oyadomari, S., Yun, C., Fisher, E.A., Kreglinger, N., Kreibich, G., Oyadomari, M., Harding, H.P., Goodman, A.G., Harant, H., Garrison, J.L., et al. (2006). Cotranslocational degradation protects the stressed endoplasmic reticulum from protein overload. Cell 126, 727–739. Romisch, K. (2005). Endoplasmic reticulum-associated degradation. Annu. Rev. Cell Dev. Biol. 21, 435–456. Song, B.L., Sever, N., and DeBose-Boyd, R.A. (2005). Gp78, a membrane-anchored ubiquitin ligase, associates with Insig-1 and couples sterol-regulated ubiquitination to degradation of HMG CoA reductase. Mol. Cell 19, 829–840. Wang, Q., Li, L., and Ye, Y. (2006). Regulation of retrotranslocation by p97-associated deubiquitinating enzyme ataxin-3. J. Cell Biol. 174, 963–971. Ye, Y., Shibata, Y., Yun, C., Ron, D., and Rapoport, T.A. (2004). A membrane protein complex mediates retro-translocation from the ER lumen into the cytosol. Nature 429, 841–847. Younger, J.M., Chen, L., Ren, H.Y., Rosser, M.F., Turnbull, E.L., Fan, C.Y., Patterson, C., and Cyr, D.M. (2006). Sequential quality-control checkpoints triage misfolded cystic fibrosis transmembrane conductance regulator. Cell126 , 571–582. 1230.e1 Cell 129, June 15, 2007 ©2007 Elsevier Inc. DOI 10.1016/j.cell.2007.06.005 .
Details
-
File Typepdf
-
Upload Time-
-
Content LanguagesEnglish
-
Upload UserAnonymous/Not logged-in
-
File Pages2 Page
-
File Size-