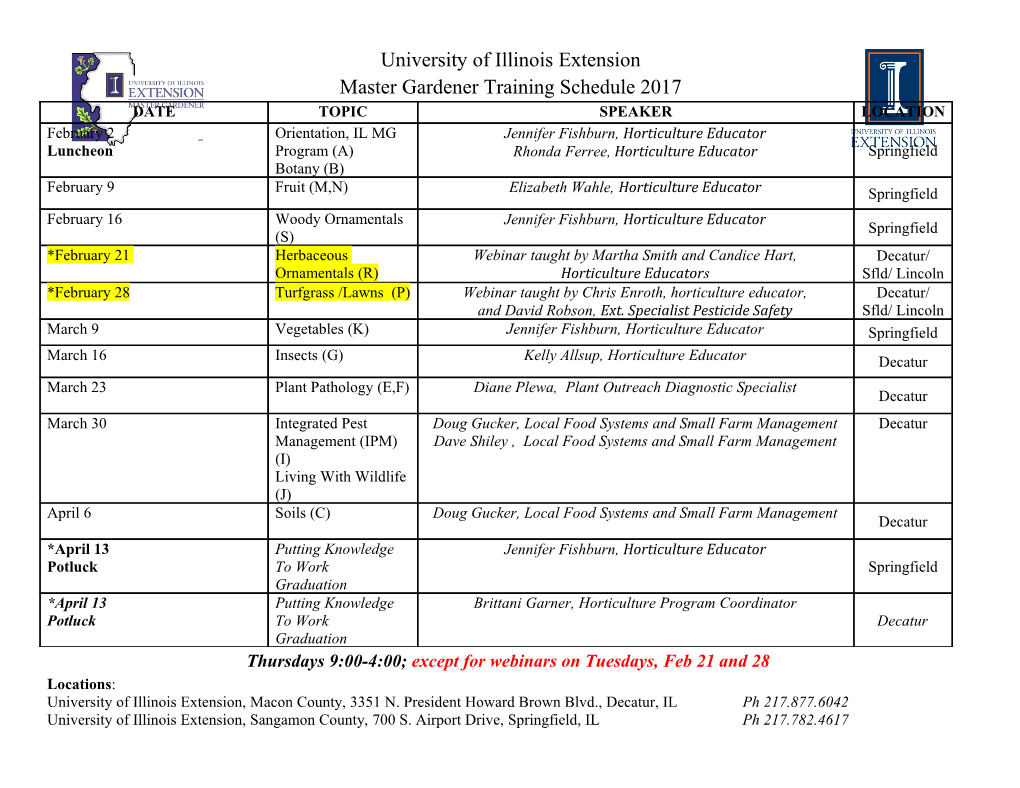
bioRxiv preprint doi: https://doi.org/10.1101/480269; this version posted November 29, 2018. The copyright holder for this preprint (which was not certified by peer review) is the author/funder. All rights reserved. No reuse allowed without permission. 1 Strategy for Efficient Importing and Function Characterizing of 2 Nicotinamide Adenosine Dinucleotide Analogs in Escherichia 3 coli Cells 4 5 Lei Wang,a Bin Liu,a Yuxue Liu,b Yue Sun,a Shanshan Zhang,a Dayu Yu,a Zongbao 6 K. Zhaob 7 8 a School of Chemical Engineering, Northeast Electric Power University, Jilin 132012, 9 China 10 b Division of Biotechnology, Dalian Institute of Chemical Physics, CAS, Dalian 11 116023, China 12 Address correspondence to Dayu Yu, [email protected], and Zongbao K. Zhao, 13 [email protected]. 1 bioRxiv preprint doi: https://doi.org/10.1101/480269; this version posted November 29, 2018. The copyright holder for this preprint (which was not certified by peer review) is the author/funder. All rights reserved. No reuse allowed without permission. 14 ABSTRACT 15 Nicotinamide adenosine dinucleotide (NAD) and its phosphorylated form (NADP) are 16 essential metabolites that are key cofactors for many redox enzymes and co-substrates 17 for several protein modification enzymes. It is difficult to study and manipulate the 18 functions of NAD(P), because these cofactors participate in complex metabolic 19 network. NAD analogs can reduce the complexity by bioorthogonalization of 20 metabolic modules and enhance our ability to understand and regulate the 21 bioenergetic and signaling pathways mediated by NAD. But the design and 22 application of NAD analogs is limited because these compounds cannot be easily 23 delivered into cells. Here we explored the strategy to import those adenine-replaced 24 NAD analogs (NXDs) into Escherichia coli cells. We showed that the transporter 25 NTT4 derived from Protochlamydia amoebophila was efficient for NXDs import. By 26 constructing an ushA-deletion mutant, we improved extracellular stability of NXDs 27 significantly and realized continuous import upon concurrent expression of NTT4. 28 The in vivo functions of NXDs were then characterized in E. coli cells. Nicotinamide 29 guanine dinucleotide was identified as an inhibitor of NAD synthesis and can partially 30 support cell growth of an NAD-auxotrophic E. coli strain. Nicotinamide cytosine 31 dinucleotide was proved an excellent energy transporter with distinct bioorthogonality 32 biocompatibility. The efficient importing system will stimulate developing and 33 screening of functional NXDs. 34 IMPORTANCE NAD analogs are importante tools for manipulating the bioenergetic 35 and signaling pathways mediated by NAD, but they have to be imported into cells as 36 these compounds are membranes impermeable. The efficiency of importing NAD 37 analogs into cells can be conspicuously improved by limiting extracellular 38 degradation while expressing an efficient NAD importer. Then the potential 39 application of the analogs can be preliminarily forecasted according to their in vivo 40 characters. That is, the analog similar to NAD is candidate regulator of NAD 41 metabolism, while analog has bare interference with natural system may serve as 42 bioorthogonal energy carrier. In summary, we explore a strategy for continuous and 43 efficient importing of NAD analogs and this work will facilitate the characterization 44 and utilization of NAD analogs. 2 bioRxiv preprint doi: https://doi.org/10.1101/480269; this version posted November 29, 2018. The copyright holder for this preprint (which was not certified by peer review) is the author/funder. All rights reserved. No reuse allowed without permission. 45 46 KEYWORDS NAD analog, nicotinamide guanine dinucleotide, nicotinamide 47 cytosine dinucleotide, cofactor manipulation, cofactor transporter, cofactor stability 3 bioRxiv preprint doi: https://doi.org/10.1101/480269; this version posted November 29, 2018. The copyright holder for this preprint (which was not certified by peer review) is the author/funder. All rights reserved. No reuse allowed without permission. 48 INTRODUCTION 49 Nicotinamide adenosine dinucleotide (NAD, Fig. 1a) and its phosphorylated form 50 (NADP) are natural pyridine nucleotide cofactors, which play a central role in biology. 51 They are essential metabolites participating in both bioenergetic and signaling 52 pathways (1, 2). But efforts to study the roles of NAD(P) have proven difficult 53 because these cofactors are subjected to tight regulation and participate in complex 54 metabolic network. Cofactor analogs are general tools for study and alteration of 55 cofactor functions, yet such efforts are limited because these compounds cannot be 56 easily delivered into cells (3-5). 57 As a pivotal class of cofactors in bioenergetic pathways, pyridine nucleotide 58 cofactors such as NAD and its reduced form NADH function as essential electron 59 acceptors or donors in numerous catabolic and anabolic reactions. They also play 60 critical roles in maintaining intracellular redox homeostasis. When modification or 61 introduction of metabolic pathways affects the level of NAD/NADP or redox state, 62 the cellular metabolism will be dramatically influenced, resulting in decreased 63 robustness and biosynthetic capacity (2, 6, 7). One promising way for avoiding 64 interference between the target cofactor dependent system and natural systems is 65 constructing bioorthogonal redox metabolic circuits based on NAD analogs. For 66 example, nicotinamide cytosine dinucleotide (NCD, Fig. 1a) wired metabolic circuits 67 can transfer energy pathway-selectively and shift the reaction equilibrium with little 68 interference with natural systems (4, 5). 69 NAD metabolism plays important roles in the maintenance of NAD pools and 70 has beneficial effects on regular calorie restriction on health span (1, 8). The 71 correlated enzymes are potential drug target for anticancer or antipathogen therapy (9, 72 10). However, characterizing the molecular mechanisms that underlie the complex 73 regulation of NAD metabolism remain unclear. Many efforts are focused on the 74 development of pyridine nucleotide cofactor analogs to serve as effective functional 75 and mechanistic probes in the cellular environment. Recombinant human 76 nicotinamide adenylyl transferase 1 (Nmnat1) was employed to condense 77 nicotinamide mononucleotide and tzATP to yield NtzAD (11). Nmnat3 could 78 synthesize nicotinamide guanine dinucleotide (NGD, Fig. 1a) (12). However, the 79 biological functions of these analogs are difficult to be investigated by exogenously 80 addition, because pyridine nucleotide cofactors are membranes impermeable (13). 81 Our ability to experimentally control in vivo specific pyridine nucleotide cofactor 4 bioRxiv preprint doi: https://doi.org/10.1101/480269; this version posted November 29, 2018. The copyright holder for this preprint (which was not certified by peer review) is the author/funder. All rights reserved. No reuse allowed without permission. 82 concentrations could enhance our understanding and application of 83 cofactor-dependent reactions. 84 Synthesis of pyridine nucleotide cofactors using corresponding membranes 85 permeable nucleosides via NAD salvage pathway is one possible route to the 86 introduction of pyridine nucleotide cofactors into cells. For example, in vivo NGD has 87 been verified in mice overexpressing Nmnat3 (13). However, recognition of 88 corresponding nucleosides by the salvage pathway may not be sufficient, and the 89 salvage pathways relying on the activation of free nucleosides to produce the desired 90 pyridine nucleotide cofactor is less than optimal (13). This will increase the challenge 91 of achieving controlled intracellular concentrations of the pyridine nucleotide cofactor, 92 which is likely to limit many applications. In general, study and application of 93 pyridine nucleotide cofactor analogs desires general means to directly introduce the 94 cofactors into cells. 95 Many NAD transporters have been discovered in both prokaryote and eukaryote 96 organisms, such as the first NAD transporter reported NTT4 derived from the 97 prokaryote chlamydial endosymbiont Protochlamydia amoebophila UWE25 (14), and 98 NAD transporters discovered in the eukaryotes Saccharomyces cerevisiae, and 99 Arabidopsis thaliana located at mitochondria or chloroplasts (12, 15). These 100 transporters import intact NAD(H) in counter exchange with ADP for cells or 101 organelles, and they are potential candidates for importing pyridine nucleotide 102 cofactor analogs. The characterization of cofactor transporters are generally carried 103 out in model organism Escherichia coli (5, 14, 16-18). Recombinant expression of the 104 NAD transporter from A. thaliana mitochondria (AtNDT2) in E. coli functions to 105 import exogenously added NCD, but it can only get 0.059 mM intracellular NCD in 106 the presence of 0.1 mM exogenous NCD (5). More efficient importing strategy is 107 desired for further application of pyridine nucleotide cofactor analogs. 108 Stability is also a key character for efficiency of cofactors import. In many 109 prokaryote and eukaryote organisms, the extracellular pyrophosphatase hydrolyzes 110 the pyrophosphate bond of pyridine nucleotide cofactors and catalyzes further 111 hydrolysis to permeable nicotinamide riboside (NR) and nucleosides (19, 20). To 112 limit or compensate extracellular decomposition of the cofactors, import of cofactors 113 is generally carried out by decreasing operating time (14, 17,
Details
-
File Typepdf
-
Upload Time-
-
Content LanguagesEnglish
-
Upload UserAnonymous/Not logged-in
-
File Pages20 Page
-
File Size-