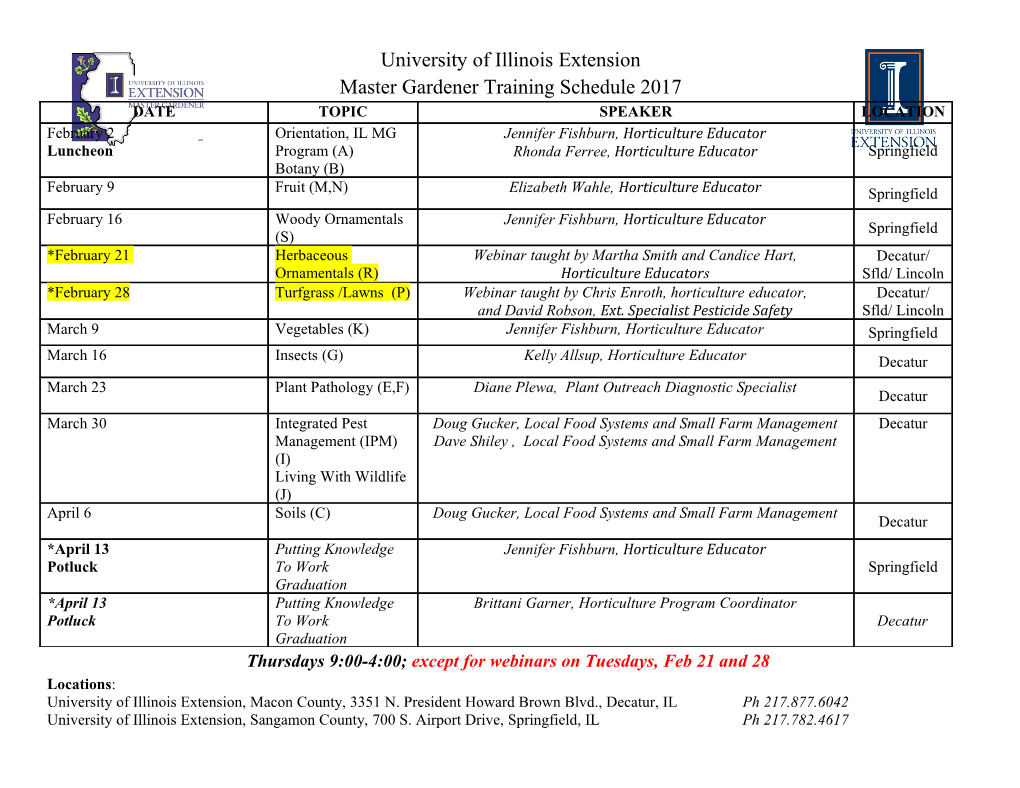
KINETIC STUDIES OF THE FOLDING OF THE MEMBRANE PROTEIN BACTERIORHODOPSIN Amjad Farooq Department of Biochemistry Imperial College London A THESIS SUBMITTED TO THE UNIVERSITY OF LONDON FOR THE DEGREE OF DOCTOR OF PHILOSOPHY 1997 ABSTRACT Stop-flow fluorescence and photodiode array spectroscopy, with a millisecond time resolution, are used here to study the refolding kinetics of the membrane protein bacteriorhodopsin. Refolding is initiated by mixing partially denatured apoprotein bacterioopsin in SDS with DMPC/CHAPS micelles. The kinetics of micelle mixing can be differentiated from the kinetics of protein folding. The results indicate that the apoprotein bacterioopsin can be refolded in the micelles to a state with a native-like secondary structure. The retinal chromophore is not required for the formation of this molten globule-like state. Further, apoprotein refolding is observed to conform to biphasic kinetics with lifetimes in the second range. This observation is taken to imply the involvement of parallel branched pathways in the refolding of the apoprotein bacterioopsin to a molten globule-like state. The mechanism of retinal binding to the apoprotein bacterioopsin is also elucidated. Two bimolecular reactions involving retinal and bacterioopsin are detected. This implies that retinal binds in a parallel fashion to the apoprotein bacterioopsin. The product of these bimolecular reactions is a spectroscopically distinct intermediate that absorbs maximally around 430 nm. The formation of this 430-nm intermediate occurs with lifetimes in the order of hundreds of milliseconds. The formation of a molten globule-like state of apoprotein is thus evidently rate-limiting for the subsequent binding of retinal. The oscillator strength of the retinal absorption band in free retinal and in the 430-nm intermediate appears to be the same. This finding is interpreted as an evidence that the retinal chromophore is non-covalently bound to the apoprotein within the 430-nm intermediate. A free energy change of - 30 kJmol-1 is determined for the formation of the 430-nm intermediate. The decay of the 430-nm intermediate into the native protein bacteriorhodopsin occurs via at least three parallel alternative routes with lifetimes ranging from several seconds to several minutes. This process is characterized by the formation of a Schiff base between the aldehyde group of retinal and the e-amino group of Lys216. A kinetic model is suggested for the folding of bacteriorhodopsin from a partially denatured state in SDS. The results of this study should have profound implications on the mechanisms of folding of proteins within biological membranes as well as in water. ACKNOWLEDGMENTS I express my sincere thanks to Jim Barber for a critical appraisal of the work, for support and advice, and for the highly comforting and productive time that I had in his laboratory whilst preparing this manuscript. I am grateful to Steve Matthews for his support and encouragement, and for the many exciting and stimulating discussions. I owe a special thanks to Jeremy Baum for his advise and support. I thank Paula Booth for her practical help with the stop-flow spectroscopy, and Martin Bell for providing the kinetic analysis software Look. I express my warm-hearted gratitude to Bee Khoo and Maria Conte for their friendship and company that I enjoyed meticulously. I offer my profound thanks to my family and, in particular, my sister Nahid, uncle Fazal and, parents Fatima and Mohammed for their understanding and appreciation. Finally, I wish to record a very special tribute to Peter Fryer (Bradford College) for it is a credit to him above all else that I have had the privilege to study for a PhD. This work was funded by the BBSRC. Amjad Farooq London 1997 CONTENTS Title 1 Abstract 3 Acknowledgments 4 Abbreviations 6 Symbols 8 List of Tables 9 List of Figures 10 Chapter 1: Introduction 15 Chapter 2: Materials and Methods 65 Chapter 3: Equilibrium Studies of Bacteriorhodopsin Refolding 91 Chapter 4: Kinetics of Bacterioopsin Refolding 111 Chapter 5: Kinetics of Retinal Binding to Bacterioopsin 129 Chapter 6: Kinetics of Retinal Binding at Various Times in the Refolding of Bacterioopsin 171 Chapter 7: Dependence of Refolding Kinetics of Bacteriorhodopsin on Retinal Concentration 189 Chapter 8: Conclusions 215 References 235 ABBREVIATIONS ANS 8-anilino-1-naphthalenesulphonate ATP adenosine triphosphate ATPase adenosine triphosphatase AU absorbance unit bO bacterioopsin bO/SDS partially denatured bO in SDS bO¢ partially refolded bO in DMPC/CHAPS bR bacteriorhodopsin BPTI bovine pancreatic trypsin inhibitor BSE bovine spongiform encephalopathy CD circular dichroism CHAPS 3-[(3-cholamidopropyl)dimethylammonio]-1-propanesulphonate CJD creutfeld-jacob disease Csp cold shock protein DHFR dihydrofolate reductase DHPC L-a-1,2-dihexanoylphosphatidylcholine DMPC L-a-1,2-dimyristoylphosphatidylcholine DNA deoxyribonucleic acid DNAse deoxyribonuclease ER endoplasmic reticulum FKBP FK506 binding protein FTIR fourier transform infra-red spectroscopy FWHM full width half maximum (of an absorption or fluorescence band) I intermediate (partially folded state) LHCII light-harvesting complex II mRNA messenger RNA N native state NMR nuclear magnetic resonance Omp outer membrane protein PAGE polyacrylamide gel electrophoresis PDA photodiode array spectroscopy PM purple membrane R all-trans-retinal RNA ribonucleic acid RNAse ribonuclease SDS sodium dodecylsulphate SRP signal recognition particle SVD singular value decomposition 2D two-dimensional 3D Three-dimensional TEMED N,N,N¢,N¢-tetramethylethylenediamine Tris Tris(hydroxymethyl)aminomethane U unfolded state UV ultraviolet SYMBOLS a experimentally observed amplitude e extinction coefficient k intrinsic rate constant K equilibrium constant l wavelength n experimentally observed rate constant R universal molar gas constant t lifetime t time T absolute temperature LIST OF TABLES Table 3.1. Comparison of chromophore absorbance and protein fluorescence maxima and their FWHM for bacteriorhodopsin refolding at pH 6 and pH 8 102 Table 3.2. Dependence of bacteriorhodopsin refolding yield on pH and the mode of retinal addition 103 Table 4.1. Comparison of experimentally observed rate constants and their amplitudes for fluorescence and absorbance refolding measurements of bacterioopsin 121 Table 5.1. Comparison of experimentally observed rate constants and their amplitudes obtained from fluorescence data for the refolding of bacterioopsin in the absence of retinal and for the reaction of retinal with bacterioopsin 152 Table 5.2. Relative oscillator strength of the retinal absorption band during the course of the reaction of retinal with bacterioopsin 154 Table 5.3. Comparison of experimentally observed rate constants obtained from fluorescence and absorbance data for the reaction of retinal with bacterioopsin 156 Table 7.1. Intrinsic rate constants, equilibrium constants and free energy changes for the phases associated with reactions involving retinal and bacterioopsin 206 Table 8.1. Folding intermediates of bacteriorhodopsin: Comparison of the various nomenclatures used in the previous studies and this thesis 223 LIST OF FIGURES Fig 1.1. A schematic diagram illustrating the concept of protein folding 18 Fig 1.2. Simplified free energy profiles of protein folding 21 Fig 1.3. Simplified energy landscapes of protein folding 28 Fig 1.4. Simplified funnels of protein folding 29 Fig 1.5. Cis-trans isomerization about proline imide bond 30 Fig 1.6. Integral membrane protein topology 33 Fig 1.7. The two-stage model of membrane protein folding 37 Fig 1.8. A simplified diagram showing a typical halobacterium 40 Fig 1.9. Chemical structure of a typical membrane lipid of halobacterium 41 Fig 1.10. Chemical structure of retinal and retinal-protein Schiff base 43 Fig 1.11. A ribbon diagram of bacteriorhodopsin 46 Fig 1.12. A diagram of bacteriorhodopsin showing amino acids lining the retinal binding pocket 47 Fig 1.13. All-trans and 13-cis configurations of retinal in bacteriorhodopsin 48 Fig 1.14. Resonance structures of the protonated Schiff base of retinal 50 Fig 1.15. Photocycle of bacteriorhodopsin 51 Fig 1.16. A general strategy for bacteriorhodopsin refolding in vitro 53 Fig 1.17. Chemical structures of SDS, CHAPS and DMPC 54 Fig 1.18. A model for the structure of mixed DMPC/CHAPS micelles 56 Fig 2.1. Absorption spectrum of all-trans-retinal in ethanol at room temperature 67 Fig 2.2. Absorption spectra of purple membrane isolated from Halobacterium salinarium and delipidated bacterioopsin 71 Fig 2.3. SDS-PAGE analysis of purple membrane and delipidated bacterioopsin 73 Fig 2.4. Stop-flow mixing circuit 78 Fig 2.5. The Xenon lamp intensity profile 80 Fig 3.1. Dependence of refolding yield of bacteriorhodopsin on bO preparation (pH 6) 94 Fig 3.2. Dependence of refolding yield of bacteriorhodopsin on the mode of retinal addition (pH 6) 97 Fig 3.3. Dependence of refolding yield of bacteriorhodopsin on the mode of retinal addition (pH 8) 100 Fig 4.1. Addition of DMPC/CHAPS to bO/SDS 114 Fig 4.2. Addition of DMPC/CHAPS to SDS 118 Fig 5.1. Addition of retinal/DMPC/CHAPS to bO/SDS 134 Fig 5.2. Addition of retinal/DMPC/CHAPS to bO/DMPC/CHAPS 144 Fig 6.1. Addition of retinal at various times in the refolding of bO 174 Fig 7.1. Bacteriorhodopsin refolding under limiting amounts of retinal 192 Fig 7.2. Bacteriorhodopsin refolding in excess retinal 198 Fig 8.1. A paradigm for bacteriorhodopsin folding 217 Fig 8.2. Energetics of Bacteriorhodopsin folding 221 IN THE
Details
-
File Typepdf
-
Upload Time-
-
Content LanguagesEnglish
-
Upload UserAnonymous/Not logged-in
-
File Pages261 Page
-
File Size-