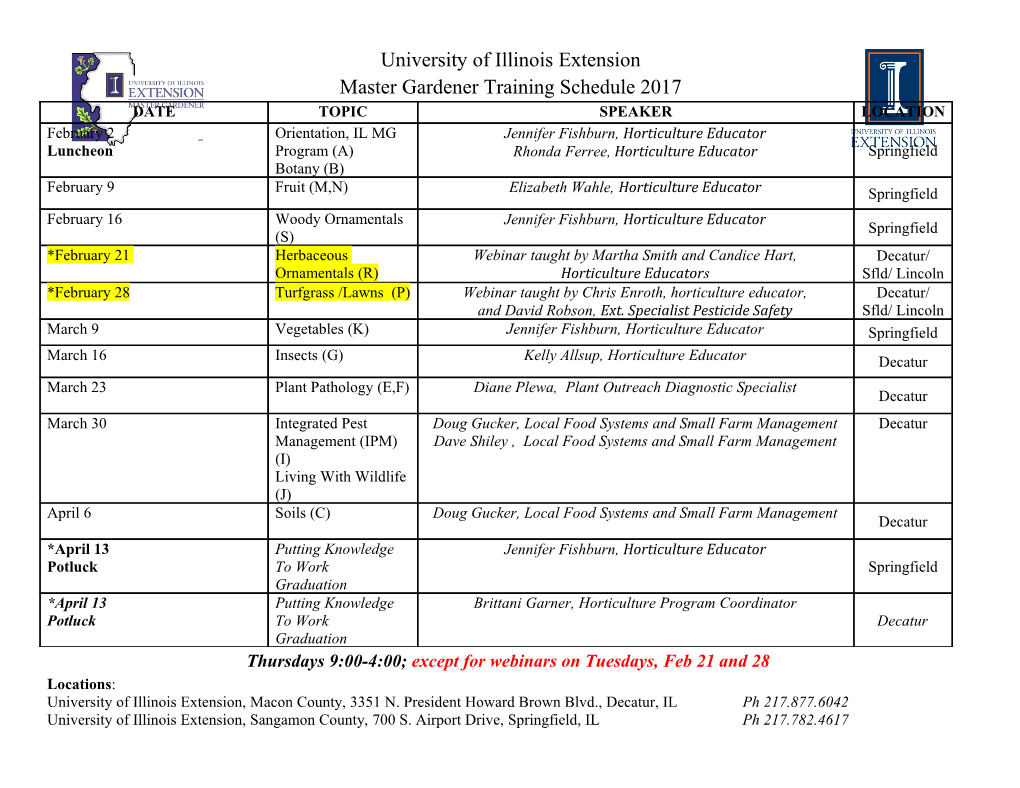
Thermophysical Analysis of Gale Crater Using Observations from TES, THEMIS and the Mars Science Laboratory Curiosity Rover Edward M. Barratt BSc (Hons), University of Edinburgh, 2011 December 5, 2013 A thesis submitted to the Faculty of the Graduate School of the University of Colorado in partial fulfillment of the requirement for the degree of Master of Science Department of Astrophysics and Planetary Science 2013 This thesis entitled Thermophysical Analysis of Gale Crater Using Observations from TES, THEMIS and the Mars Science Laboratory Curiosity Rover written by Edward Mark Barratt has been approved for the Department of Astrophysical and Planetary Science Prof. Brian Hynek Signature Date Dr. Nathaniel Putzig Signature Date Prof. Shijie Zhong Signature Date Dr. Mike Mellon Signature Date The final copy of this thesis has been examined by the signatories, and we find that both the content and the form meet acceptable presentation standards of scholarly work in the above mentioned discipline. ii Edward Mark Barratt (Masters of Science, Department of Astrophysics and Planetary Science) Thermophysical Analysis of Gale Crater Using Observations from TES, THEMIS and the Mars Science Laboratory Curiosity Rover Thesis directed by Professor Brian Hynek of the department of Geological Sciences. Abstract I created a web-based interface to the MARSTHERM one-dimensional numerical thermal model, which cal- culates surface and atmospheric temperatures of Mars for a set of user-specified conditions. The website also provides access to tools which I adapted to allow users to automatically derive thermal inertia from images taken by the Mars Odyssey Thermal Emission Imaging System (THEMIS), and access to existing maps of thermal inertia derived from Mars Global Surveyor Thermal Emission Spectrometer (TES) observations. To demonstrate the capabilities of the tools provided on the website, I conducted a case study investigating the thermal inertia within Gale Crater, using observations from TES, THEMIS, and the Mars Science Lab- oratory (MSL) Ground Temperature Sensor (GTS). Seasonal variations in TES-derived thermal inertia in the vicinity of the MSL landing site are consistent with a layer of sand or dust over rock, an interpretation supported by diurnal temperature variations recorded by GTS on MSL sol 30. However, diurnal tempera- ture variations from elsewhere along the MSL traverse route could not be modeled by simple two-component structures. Seasonal variations in TES-derived thermal inertia support the hypothesis that the thickness of a surface layer of dust likely increases with elevation on Mount Sharp. iii Acknowledgments None of this work would have been accomplished without the advice, patience and assistance of Than Putzig at the Southwest Research Institute, who generously entrusted me with the construction of the MARS- THERM website whilst supporting and encouraging my research efforts. I am also grateful for the guidance and hard work of Brian Hynek and Fran Bagenal, who both assisted me in navigating the complications of educational bureaucracy and were quick to offer advice when I needed it. Thanks are also due to the other members of my committee, Mike Mellon and Shijie Zhong. And also to my good friends and housemates at the Mackshack for making the last two years in Boulder so enjoyable. iv Contents List of Figures vii List of Tables viii 1 Introduction 1 2 The MARSTHERM Thermal Model 5 2.1 Thermal Inertia Derivation . 7 2.1.1 Single Point Thermal Inertia Derivation . 7 2.2 Surface Heterogeneity . 8 2.3 Instruments . 11 2.3.1 TES . 11 2.3.2 THEMIS . 11 2.3.3 REMS . 12 2.3.4 MOLA . 13 2.3.5 Imaging Instruments . 13 3 The MARSTHERM Website 14 3.1 Project Designation . 16 3.1.1 Project Maps . 16 3.1.2 Dust Opacity . 16 3.1.3 Two Component Modeling . 17 3.2 THEMIS Image Processing . 17 3.2.1 Download and Pre-Processing . 17 3.2.2 Thermal Inertia Derivation . 19 3.2.3 Output Files . 20 3.2.4 Known Issues . 21 4 Gale Crater 26 4.1 Mount Sharp . 26 4.1.1 Thermal Inertia of Mount Sharp . 28 4.2 Northern Crater Floor . 29 v 4.2.1 Peace Vallis and Peace Vallis Fan . 32 4.3 Diurnal Temperature Cycles . 42 4.3.1 MSL sol 30 . 43 4.3.2 MSL sol 100 . 46 5 Conclusions 50 6 References 52 vi List of Figures 1 Seasonal atmospheric pressure within Gale Crater. 3 2 Thermal inertia and particle-size scaling. 4 3 Model diurnal surface temperatures at lookup-table nodes. 9 4 THEMIS image times and seasons for Gale Crater. 12 5 MARSTHERM website and model temperatures in Gale Crater. 15 6 TES dust opacity history within Gale Crater. 19 7 THEMIS thermal inertia products for Gale Crater from the MARSTHERM website. 22 8 Histogram of global TES-derived thermal inertia values. 23 9 Simulated Histogram showing Shark-fin Artifacts. 25 10 Gale Crater THEMIS visible mosaic. 27 11 THEMIS brightness temperature and derived thermal inertia for Gale Crater. 30 12 THEMIS brightness temperature and derived thermal inertia for a profile across Mount Sharp. 30 13 TES-derived thermal inertia of Mount Sharp. 31 14 Seasonal variations in apparent thermal inertia on Mount Sharp. 31 15 Peace Vallis thermal inertia. 33 16 Peace Vallis elevation from HRSC DTM. 34 17 Histograms of Peace Vallis thermal inertia. 35 18 The high thermal inertia area of the Peace Vallis fan. 36 19 Histograms of thermal inertia in the Peace Vallis fan. 37 20 HiRISE images of the high thermal inertia area of the Peace Vallis fan. 38 21 Annual-median TES-derived thermal inertia on the Peace Vallis fan. 39 22 Seasonal variations in apparent thermal inertia on the Peace Vallis fan. 41 23 HiRISE image of the Curiosity traverse. 42 24 Mosaic of MSL sol 30 site. 44 25 GTS diurnal temperature cycle at MSL sol 30 site. 45 26 Left navigation camera image of MSL sol 100 site at Rocknest. 47 27 GTS diurnal temperature cycle at MSL sol 100 site, with single component model curves. 48 28 GTS diurnal temperature cycle at MSL sol 100 site, with two component model curves. 49 vii List of Tables 1 Thermal model parameters. 6 2 Thermal model lookup-table properties. 8 3 Idealized surface materials. 10 4 Apparent thermal inertia of Peace Vallis Fan. 40 5 MSL diurnal temperature cycles. 43 viii 1 Introduction Since 1996, when Mars Global Surveyor (MGS) began the first successful mission to Mars since the Viking era, Mars has been the target of 9 successful missions. Two rovers are currently exploring the surface of the planet, and three orbiters are in place continually gathering and returning data. With still more missions planned by NASA, ESA, and the Russian and Indian space agencies, no other planet apart from our own has ever been subject to such intense and active exploration. These missions have revealed clear evidence that the past climate of Mars was very different from how it is now. With newer data the sparse valley networks observed by the Viking orbiters (e.g. Masursky et al. 1977) have been revealed as integrated and mature drainage systems indicative of flowing surface water (Hynek et al. 2010). Spectral data indicates that clay minerals, which only form in aqueous conditions, occur in sedimentary rocks on the surface (e.g. Michalski et al. 2005; Milliken et al. 2010). The global distribution of deltas and valleys even suggests the possibility of an ancient Martian ocean (Di Achille & Hynek 2010). All of this evidence provides proof that Mars was at one time warmer and wetter than it is now. Understanding more about these early conditions can help us to theorize about the habitability of the early planet. Could Mars have once hosted life? And if so, are there signs that it did? Continued research on the geology of Mars can help to answer these questions, while answers to them will also help us to understand the nature of life on our own planet. This knowledge will provide us with a better idea of past or present conditions that may support life on other planets in our own solar system and beyond. While rovers provide a means of investigating the geology of the surface in situ at a few small sites, investigations over broader regions are predominantly limited to using data from remote sensing instruments on the Martian orbiters or Earth based telescopes. Surface temperature is one measurement that can be derived from remote-sensing observations. The temperature of a planetary surface is controlled by a balance of the outward radiated heat from the surface with the inward heat flux due to solar insolation (SM ) and − atmospheric radiation (FIR), while also accounting for heat exchange with the subsurface by conduction, and any other heat sources. In the case of Mars, another heat source that must be considered is latent heat due to seasonal CO2 condensation and sublimation. This balance provides an equation for the surface temperature (Ts) (e.g. Mellon et al. 2008): r 4 − @m π @T σTs = SM (1 − A) cos i + FIR + L + I 0 (1) @t Γ @Z Z0=0 where is the surface emissivity, σ is the Stefan-Boltzmann constant, A is the albedo, i is the solar incidence 1 angle and L is the latent heat of CO2 sublimation or condensation, and m is the mass of CO2 frost. I is the thermal inertia, Γ is the seasonal (or diurnal) period, and T is the subsurface temperature while Z0 is depth normalized by the seasonal (or diurnal) thermal skin depth δ, where δ is given by: r I Γ δ ≡ (2) ρc π where ρ is the density and c is the heat capacity of the surface materials.
Details
-
File Typepdf
-
Upload Time-
-
Content LanguagesEnglish
-
Upload UserAnonymous/Not logged-in
-
File Pages65 Page
-
File Size-