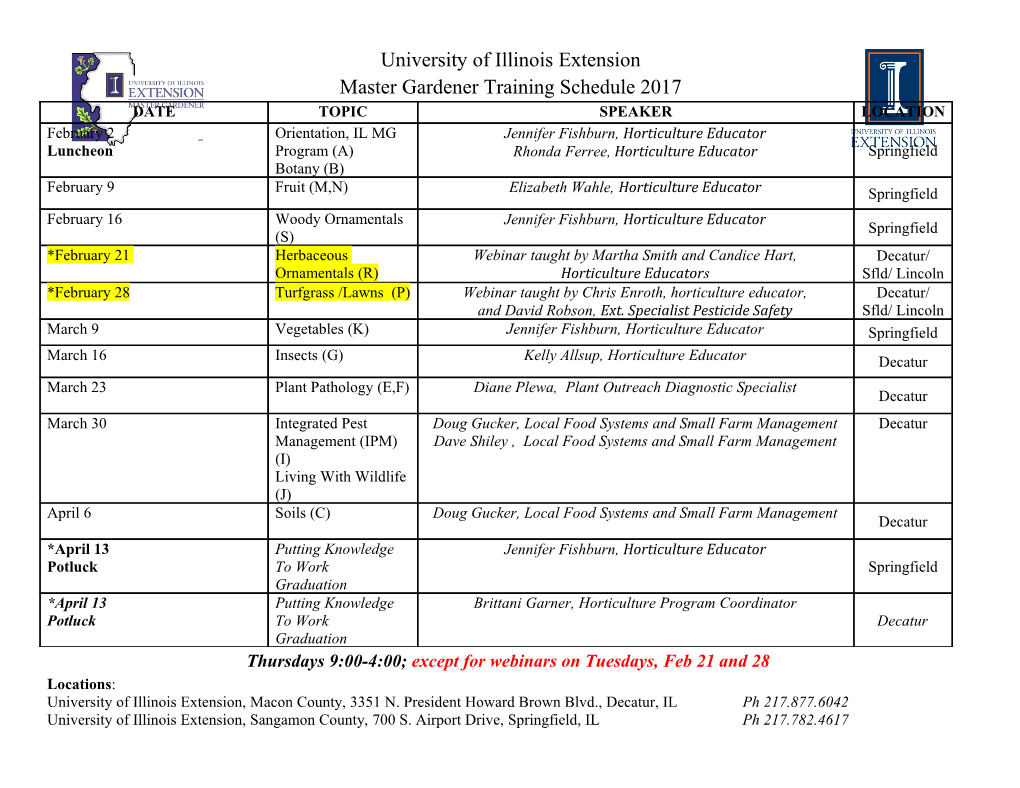
Biaxial Testing of Sheet Metal: An Experimental-Numerical Analysis Gerard Quaak MT 08.10 TU/e Master Thesis May, 2008 Engineering thesis committee prof.dr.ir. M.G.D. Geers (Chairman) dr.ir. J.P.M. Hoefnagels (Coach) ir. C. Tasan (Coach) dr.ir. P.J.G. Schreurs dr.ir. H. Vegter Eindhoven University of Technology Department of Mechanical Engineering Computational and Experimental Mechanics Abstract With the recent increase in the popularity of advanced high strength steels (e.g. dual phase, TRIP) in automotive industry, new challenges have arisen. Conventional continuum models are found not to capture the reported pre- mature ductile failures in such steels, which are governed by damage evolution. Another challenge is understanding and predicting metal behaviour under com- plex strain paths. The ability to precisely capture these eects in continuum models is important for the sheet metal forming industry, in order to carry out these processes as ecient as possible. However, improvements of the numerical tools highly depend on the development of accurate and practical experimental techniques. A testing device for biaxial deformation of sheet metal is such an experimental tool that has been studied by many researchers before. Although several experimental set-ups have been proposed in the literature, most of these designs are not capable of providing information up to the point of fracture. Additionally, these set-ups are not usable for real-time, in-situ examination of the deforming structure with advanced microscopic techniques such as SEM, AFM, surface prolometry, or digital image correlation, because a miniaturized form is not available or was never investigated. The main goal of this project therefore is to nd a practical and accurate set- up that can deform sheet metal specimens under varying complex strain paths, while allowing for real-time, in-situ microscopic examination. For this pur- pose, several experimental set-ups (e.g. bulge, punch, Marciniak and cruciform tests) have been studied and compared both experimentally and numerically. For testing cruciform samples a simplied in-plane biaxial-loading set-up was designed and build, while Marciniak tests were carried out at Corus RD&T. The corresponding experimental results were used to verify and compare these tests in terms of practical aspects (e.g. specimen preparation). The computa- tional results are used to analyze stress and strain distributions and for better understanding of the eects of miniaturization. Combining literature, numerical and experimental test results, it was concluded that the cruciform and Marciniak test are the most promising set-ups for minia- turized biaxial testing of sheet metal with in-situ microscopic examination. Both test have their limits, but when taken these into account can provide valuable data. i Samenvatting Met de recente populariteit van 'advanced high strength' staalsoorten (dual phase, TRIP) in de automotive industrie, zijn nieuwe vraagstukken ontstaan. Conventionele continuum modellen blijken niet in staat om de aanwezige taaie breuk te beschrijven, waardoor het voorspellen van het materiaal gedrag tijdens omvormen van deze metalen niet mogelijk blijkt. Wanner niet lineaire rek paden een rol spelen blijken de huidige continuum modellen zelfs nog minder accuraat. Voor het verbeteren van de numerieke gereedschappen zijn echter betrouwbare experimentele technieken nodig, zodat het materiaal gedrag voor deze groep metalen is te bepalen. Een voorbeeld van zo een opstelling, is er een om biaxiale deformatie van plaat staal te bestuderen. Hiernaar is reeds veel onderzoek aan besteed, maar hoewel verschillende gereedschappen onderzocht zijn, bestaat er tot op heden geen opstelling om tot breuk te deformeren zonder externe invloeden. Daar komt nog bij dat om de micro structuur van het materiaal te onderzoeken geavanceerde technieken als SEM en AFM of digital image correlation gebruikt moeten worden, wat inhoudt dat een miniatuur opstelling nodig is. Mogelijkheden voor een dergelijke opstelling zijn nog niet eerder onderzocht. Het belangrijkste doel van dit project is dan ook het vinden van een praktische manier om plaat staal onder veranderende rek paden, met de mogelijkheid real- time metingen te doen. Hiervoor zijn verschillende experimentele opstellingen (bulge, punch, Marciniak en cruciform test) numeriek en experimenteel onder- zocht en vergeleken. De numerieke resultaten geven o.a. inzicht in spannings- en rek velden en de eecten van miniaturisatie, de experimentele resultaten worden gebruikt voor vericatie en vergelijk op praktische gebied, zoals het maken van test samples. Voor het testem van kruisvormige trekstaven is een opstelling ontworpen en gebouwd, als onderdeel van dit onderzoek. Voor de Marciniak testen is gebruik gemaakt van een opstelling beschikbaar gesteld door Corus RD&T. De literatuur studie, numerieke en experimentele resultaten laten zien dat zowel de kruisvormige trekstaven als de Marciniak test bruikbaar zijn in een geminia- turiseerde vorm. Beide tests hebben beperkingen, maar wanneer hier rekening mee wordt gehouden kunnen zij waardevolle informatie opleveren. ii Acknowledgement This Master project was mainly carried out at Eindhoven University of Tech- nology, in the group of professor Marc Geers. Experimental work has been done at Corus RD&T in IJmuiden and in the University Multi-scale Labora- tory. Special thanks goes to Sjef Garenfeld for his time and patience in the design process of the biaxial testing set-up and specimens. I also want to thank Marc van Maris, supervisor of the Multi-scale Laboratory, for his guidance and advice during experimental work and Tom Engels for his help with the tensile testing machine. From I want to thank Corus RD&T Menno de Bruine, oper- ator of the Marciniak test set-up, Carel ten Horn and Louisa Carless for their time and eort with the experimental work on the Marciniak test. At Philips Drachten a lot of insight was provided in possible material removal techniques, for which I want to express my gratitude to Gerrit Klaseboer, Harmen Altena and Willem Hoogsteen for receiving us and a good discussion of the material removal problem. I also want to thank Johan Hoefnagels and Cem Tasan for their input in the project, the many evenings discussing and the help with the nal report. Gerard Quaak Eindhoven, May 2008 iii Contents Abstract i Samenvatting ii 1 Introduction 1 1.1 Formability and strain path dependency . 2 1.2 Objective . 4 1.3 Strategy . 4 2 Literature Survey 5 2.1 Introduction . 5 2.2 Bulge test . 8 2.3 Punch test . 11 2.4 Marciniak test . 14 2.5 In-plane loading with cruciform geometry . 17 2.6 Summary . 22 3 Numerical methodology 23 3.1 Material model . 23 3.2 Bulge test . 24 3.3 Punch test . 25 3.4 Marciniak test . 27 3.5 In-plane loading with cruciform geometry . 28 4 Experimental methodology 29 4.1 Marciniak test . 29 4.1.1 In-plane testing with the Marciniak set-up . 29 4.1.2 Specimen manufacturing . 30 4.2 In-plane loading with cruciform geometry . 30 4.2.1 Design of a test set-up . 30 4.2.2 Tests with in-plane cruciform geometry . 34 4.2.3 Specimen manufacturing and characterization . 36 5 Results 39 5.1 Bulge test . 40 5.1.1 Miniaturization . 40 5.1.2 Summary . 42 5.2 Punch test . 43 iv CONTENTS 5.2.1 Miniaturization . 44 5.2.2 Summary . 46 5.3 Marciniak test . 47 5.3.1 Working principle of the Marciniak test . 47 5.3.2 Numerical - experimental study of the Marciniak test . 49 5.3.3 Minaturization . 53 5.3.4 Summary . 55 5.4 In-plane loading with cruciform geometry . 56 5.4.1 Optimization of the cruciform design . 56 5.4.2 Proof of principle . 57 5.4.3 Specimen manufacturing and characterization . 60 5.4.4 Miniaturization . 66 5.4.5 Summary . 67 5.5 Comparative evaluation of the set-ups . 68 6 Conclusions and recommendations 72 6.1 Conclusions . 72 6.2 Recommendations for future work . 73 Bibliography 75 A Electrical Discharge Machining 79 B Electrical Chemical Machining (ECM) 83 C Specimen Preparation: TegraPol or Target System 86 v Chapter 1 Introduction Metals, and in particular sheet metals, are used in a wide variety of appli- cations in industry, with the main elds of application being packaging (food containers, beverage cans), automotive and aerospace industry. As material costs are a substantial part of the costs of manufactured products and most of the products are produced in large numbers, large cost reductions can be achieved by lowering the amount of material used. Moreover, several other reasons that can be thought of for lowering the amount of used material, e.g. lowering weight and lowering impact on the environment by polluting. All of these reasons result in eorts to achieve material use reduction without quality loss in the product. Figure 1.1: Advantages and disadvantages of using aluminium instead of steel for a standard medium size car [44] In the automobile industry a rst attempt to achieve weight reduction was done by using low density materials like aluminium, magnesium and plastics, but recent studies show a promising future for steels instead. The International Iron and Steel Institute (IISI) computed how the use of aluminium makes the car body lighter, but does not have a signicant eect on the total weight of the car and causing more environmental impact because of higher equivalent CO2-emissions (see gure 1.1). The development of Advanced High Strength Steels that can replace the existing steels is therefore closely followed by the automotive industry. [16, 44] 1 CHAPTER 1. INTRODUCTION Figure 1.2: Overview of steel grades as used in the automotive industry [44] Figure 1.2 shows an overview of regularly used types of steel for automotive applications. In this gure the materials furthest to the left are most suitable for forming virtually any desired shape, while consuming relative low amounts of energy.
Details
-
File Typepdf
-
Upload Time-
-
Content LanguagesEnglish
-
Upload UserAnonymous/Not logged-in
-
File Pages94 Page
-
File Size-