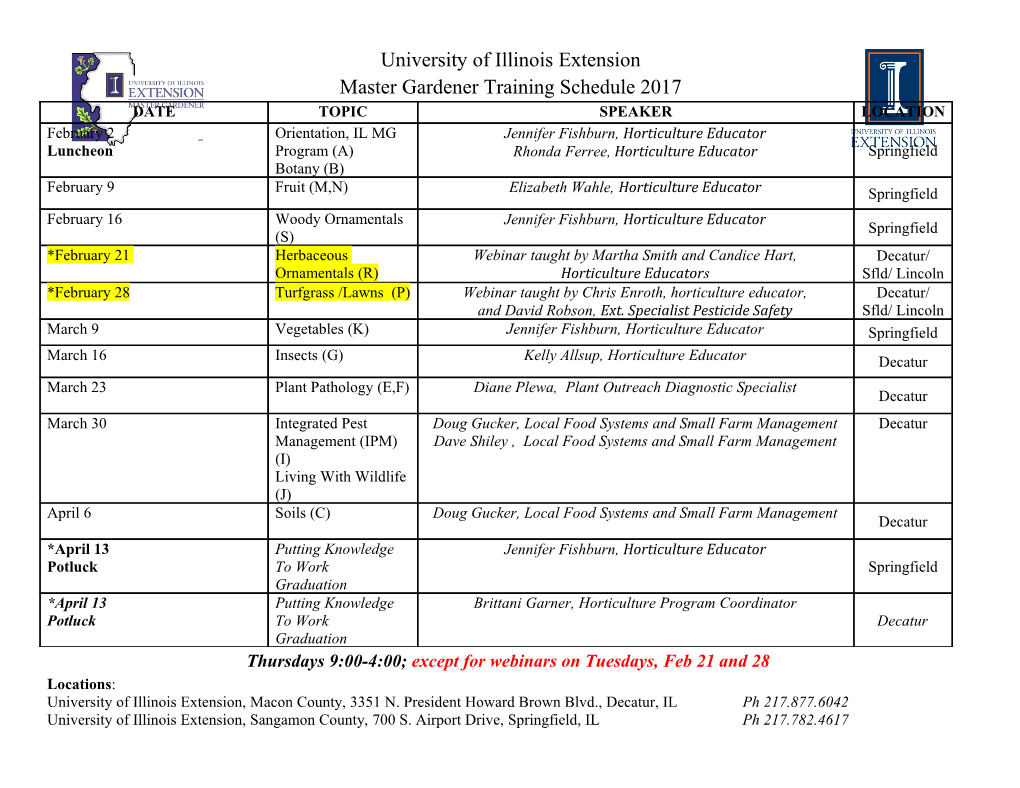
Hydrogen-bonding networks and RNA bases revealed by cryo electron microscopy suggest a triggering mechanism for calcium switches Peng Gea,b,c and Z. Hong Zhoua,b,c,1 aDepartment of Microbiology, Immunology, and Molecular Genetics and the bCalifornia NanoSystems Institute, University of California, Los Angeles, CA 90095-7364; and cStructural Computational Biology and Molecular Biophysics Program, Baylor College of Medicine, Houston, TX 77030 Edited* by Juli Feigon, University of California, Los Angeles, CA, and approved April 14, 2011 (received for review December 8, 2010) Helical assemblies such as filamentous viruses, flagella, and F-actin TMV has a chemical switch that causes the protein to change represent an important category of structures in biology. As the first when the environment changes. CP has several clusters of acidic discovered virus, tobacco mosaic virus (TMV) was at the center amino acids (including a cluster of negative charges formed by an of virus research. Previously, the structure of TMV was solved at aspartic acid residue and a phosphate moiety of the viral RNA) atomic detail by X-ray fiber diffraction but only for its dormant or that are stable outside of cells, where calcium-ion and proton high-calcium-concentration state, not its low-calcium-concentration levels are relatively high, but repel one another in the low-calcium, state, which is relevant to viral assembly and disassembly inside low-proton conditions inside cells. CP mediates assembly and host cells. Here we report a helical reconstruction of TMV in its disassembly through these carboxyl-carboxylate or phosphate- carboxylate pairs that switch states at different calcium and proton calcium-free, metastable assembling state at 3.3 Å resolution by concentrations (11, 13, 14). Such a regulation is common in plant cryo electron microscopy, revealing both protein side chains and viruses. Each of these clusters chelates a calcium ion at high- RNA bases. An atomic model was built de novo showing marked calcium concentration or shares a proton at high-proton concen- differences from the high-calcium, dormant-state structure. Al- tration; and, due to their negative charges, amino acid residues though it could be argued that there might be inaccuracies in the within each of these clusters repulse one another in the absence of latter structure derived from X-ray fiber diffraction, these differen- a calcium ion or proton. Previously, a 2.9 Å resolution structure of ces can be interpreted as conformational changes effected by TMV in high-calcium concentration was solved by X-ray fiber calcium-driven switches, a common regulatory mechanism in plant diffraction (6). In contrast, TMV is relatively poorly understood in viruses. Our comparisons of the structures of the low- and high- its low-calcium state. TMV structures in its calcium-free confor- calcium states indicate that hydrogen bonds formed by Asp116 mation have been determined by cryoEM at 9 Å (15) and 4.7 Å and Arg92 in the place of the calcium ion of the dormant (high- (9). However, the limited resolutions in these structures have calcium) state might trigger allosteric changes in the RNA base- precluded the identification of protein–RNA interactions neces- binding pockets of the coat protein. In turn, the coat protein–RNA sary to establish the mechanism of viral assembly. interactions in our structure favor an adenine-X-guanine (A*G) mo- The high redundancy intrinsic to helical objects makes them tif over the G*A motif of the dormant state, thus offering an expla- good subjects for cryoEM averaging. However, no helical cryoEM nation underlying viral assembly initiation by an AAG motif. structures determined to date have surpassed the 4 Å barrier, thus prohibiting de novo full-atom model building. Examples include the nicotinic acetylcholine receptor (16) and the bacterial flagellar elical assemblies represent a very important class of biological filament (17), structures of which have reached but not surpassed Hstructures, performing various tasks for the proper functions 4 Å resolution. Limitations in both instrumentation and data of life or executing malicious functions in disease. For example, processing may have contributed to this resolution barrier. On the protein helical assemblies such as cytoskeletal networks and one hand, magnification variability due to nonparallel illumination muscle fibers are essential components in our bodies (1, 2). Other fl and phase errors due to beam tilt can severely limit the resolution helical assemblies include bacterial agella and secretion systems. of the cryoEM structures obtained. Recently, techniques such as Helical viruses, such as tobacco mosaic virus (TMV), account for parallel illumination and coma-free alignment have been used in a major fraction of the virus kingdom. Such helical structures, cryoEM (18, 19), eliminating many of the resolution barriers im- bearing a special kind of 2D periodicity and potential flexibility, are fi posed by previous imaging systems. On the other hand, previous dif cult for structural determination to atomic resolution by con- helical reconstruction methods have demanded “perfect” helical ventional methods including X-ray crystallography and NMR. symmetry for higher-resolution reconstructions, a condition that Thus, the molecular mechanisms of action in many of these sys- cannot be met by helical assemblies in reality. The iterative helical tems are not understood to atomic detail. Even for TMV, the first real-space reconstruction method (IHRSR) (20) has made it MICROBIOLOGY virus ever isolated and extensively studied (3) by both X-ray dif- – possible to deal with such imperfections. fraction (e.g., 4 8) and cryo electron microscopy (cryoEM) (9, 10), Here we take advantage of these latest technical developments we still do not know its structure at atomic detail in a state that is and further improve the helical reconstruction method. By doing relevant to its assembly and disassembly processes, and the un- so, we have determined the structure of TMV in its metastable derlying mechanisms of these processes remain unknown. calcium-free state at 3.3 Å resolution by cryoEM helical re- The viral RNA of TMV is infectious by itself, but RNA on its construction. Our structure reveals the mechanisms by which the own is very unstable. The addition of a 17-kDa coat protein (CP) around the RNA protects the RNA from degrading and makes TMV very stable. The assembly of a rod-shaped virion begins at a two-turn helical CP complex, called the 20S aggregate (11), which Author contributions: P.G. and Z.H.Z. designed research; P.G. performed research; P.G. recognizes the initiation motif. This motif contains an AAG repeat and Z.H.Z. analyzed data; and P.G. and Z.H.Z. wrote the paper. (11), with the last G being the most conserved (12). Each mature The authors declare no conflict of interest. TMV is composed of 2,130 CP molecules and a 6-kb linear posi- *This Direct Submission article had a prearranged editor. tive-strand RNA, organized in a helical rod of 3,000 Å in length Data deposition: The cryoEM density map and the atomic model have been deposited and 180 Å in diameter. With the protection of CP, TMV can in the EMDB and Protein Data Bank (ID codes EMD-5185 and 3J06, respectively). survive for years in cigars and cigarettes made from infected leaves. 1To whom correspondence should be addressed. E-mail: [email protected]. The protein coat poses a problem, however, because CP must This article contains supporting information online at www.pnas.org/lookup/suppl/doi:10. be removed once the virus gets inside a cell. As for many viruses, 1073/pnas.1018104108/-/DCSupplemental. www.pnas.org/cgi/doi/10.1073/pnas.1018104108 PNAS | June 7, 2011 | vol. 108 | no. 23 | 9637–9642 Downloaded by guest on September 27, 2021 TMV coat protein swaps base favorability (G*A to A*G) in re- S2A). In our strategy, projection generation and matching are only sponse to the removal of calcium ions from its calcium switches. performed at azimuthal angle locations 0, 1θ − 1δ,2θ − 2δ...until Our structure also explains why both assembly and disassembly 360° is reached, in which (θ − δ)° is the size of an actual angular step can happen with the same components in the same environment. (which is the angular difference between two neighboring projec- tions within the same altitude) and where θ is an arbitrarily chosen Results angle that is much larger than the desired angular interval δ.(Due Determining Helical Structure to Atomic Resolution. As detailed in to space limitations, a brief description is provided below. For more Methods, we optimized the acquisition of high-resolution images detailed guidelines, see SI Discussion.) in three aspects. First, we used a thoroughly cleaned and exten- We first find the upper limit of δ based on the targeted reso- sively “baked” holey carbon grid as well as a small beam size to lution and the Crowther formula (21). For the case of TMV, δ has minimize specimen charging. Second, we used parallel illumination to be less than 1° to achieve 3.3 Å resolution; it also has to be an ϕ to eliminate magnification differences across images at different integral fraction of the angular span of the asymmetric unit, (in ϕ defocus settings. Third, we minimized beam tilt to reduce phase the case of TMV, = 22.04°), for the vernier strategy to work. δ ϕ ϕ errors. Electron micrographs of frozen hydrated TMV in the meta- Therefore, the available choices of for TMV here are /22, /23, ϕ stable calcium-free state show characteristic striations perpendic- /24, and so forth. Then we experiment on different combinations θ δ θ ular to the helical axes (Fig. 1A). These particles were boxed into of and that satisfy the following three relationships: First, is A an integral fraction or an integral multiplicity of ϕ (i.e., ϕ =mθ); segments (see box in Fig.
Details
-
File Typepdf
-
Upload Time-
-
Content LanguagesEnglish
-
Upload UserAnonymous/Not logged-in
-
File Pages6 Page
-
File Size-