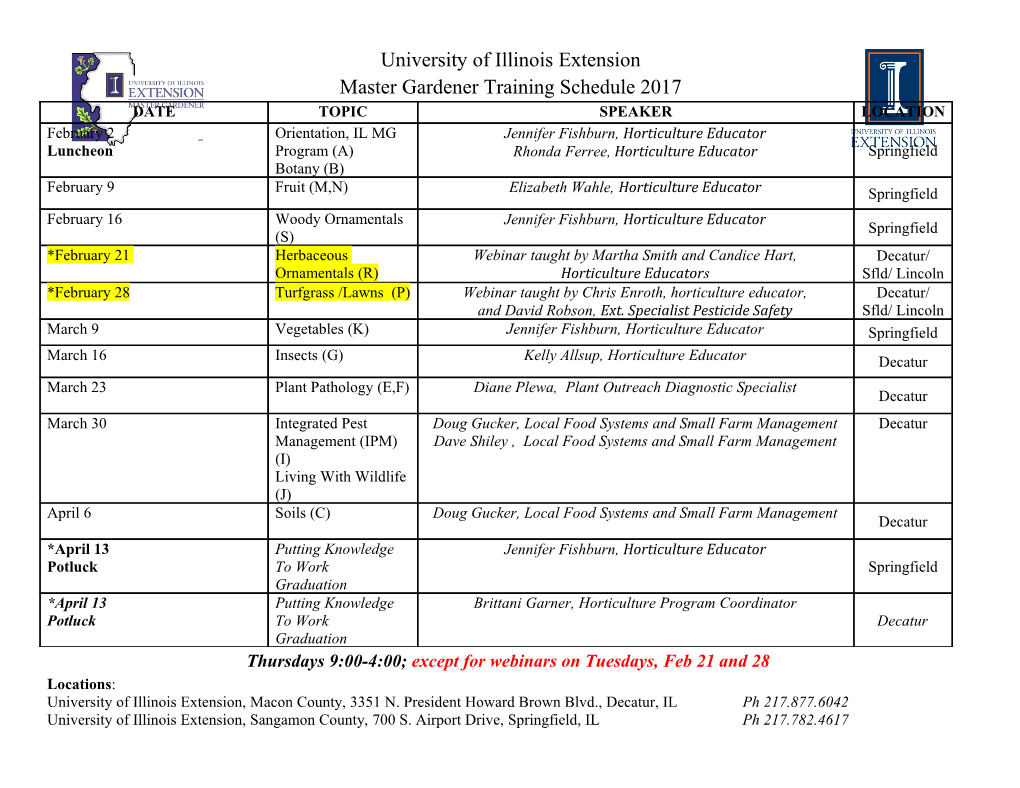
A RESONANT CAPACITIVE TEST STRUCTURE FOR BIOMOLECULE SENSING Thesis Submitted to The School of Engineering of the UNIVERSITY OF DAYTON In Partial Fulfillment of the Requirements for The Degree of Master of Science in Electrical Engineering By Danielle Nichole Bane UNIVERSITY OF DAYTON Dayton, Ohio August, 2015 A RESONANT CAPACITIVE TEST STRUCTURE FOR BIOMOLECULE SENSING Name: Bane, Danielle Nichole APPROVED BY: Guru Subramanyam, Ph.D. Karolyn M. Hansen, Ph.D. Advisor Committee Chairman Advisor Committee Member Professor and Chair, Department of Professor, Department of Biology Electrical and Computer Engineering Partha Banerjee, Ph.D Committee Member Professor, Department of Electrical and Computer Engineering John G. Weber, Ph.D. Eddy M. Rojas, Ph.D., M.A., P.E. Associate Dean Dean School of Engineering School of Engineering ii c Copyright by Danielle Nichole Bane All rights reserved 2015 ABSTRACT A RESONANT CAPACITIVE TEST STRUCTURE FOR BIOMOLECULE SENSING Name: Bane, Danielle Nichole University of Dayton Advisors: Dr. Guru Subramanyam and Dr. Karolyn M. Hansen Detection of biomolecules in aqueous or vapor phase is a valuable metric in the assessment of health and human performance. For this purpose, resonant capacitive sensors are designed and fab- ricated. The sensor platform used is a resonant test structure (RTS) with a molecular recognition element (MRE) functionalized guanine dielectric layer used as the sensing layer. The sensors are designed such that the selective binding of the biomarkers of interest with the MREs is expected to cause a shift in the test structure’s resonant frequency, amplitude, and phase thereby indicating the biomarker’s presence. This thesis covers several aspects of the design and development of these biosensors. Guanine biopolymer is characterized using capacitive test structures (CTSs) and RTSs with guanine dielectric layers. From this characterization, the dielectric constant and loss tangent of guanine are found to be 5.345 ± 0.294 and 0.015 ± 0.001 respectively. The resonance of the RTS with guanine dielectric layer is 3.148 ± 0.079 GHz with a notch depth of 7.472 ± 0.330 dB. To further characterize guanine, contact angle measurements with water were performed to deter- mine the hydrophobic/hydrophilic properties. The contact angle is 62.07◦ ± 3.029◦ indicating the guanine thin films are slightly hydrophobic in comparison to glass (contact angle is 41.4◦ ± 2.72◦). Additionally, a chemical functionalization method for guanine is developed. In this method, a cross- linker is simultaneously and covalently bound to the surface of the guanine and to the biomolecule iii thereby creating a covalent tether. Tests employing a biotin-streptavidin model indicate the chemical functionalization method is viable. In addition to the resonant capacitive sensor, two radio frequency (RF) test structures are devel- oped: an RF bridge and a half-wavelength resonator. Both of these test structures have gaps in the transmission lines that will be bridged with MRE functionalized carbon nanotubes (CNTs). Any binding event between an analyte of interest and MRE is expected to cause a shift in the resonance of the test structures. The test structures are designed for a resonance at 8 GHz and are simulated in Applied Wave Research Design Environment (AWR) software. For the RF bridge, the simulation’s resonance is at 9.2 GHz with a notch depth of 20.31 dB. The simulation of the half-wavelength resonator shows a resonance at 8 GHz with a pass band peak of 0.3395 dB. Additionally, the RF bridge test structure is fabricated. Measurements pre-CNT integration reveal a resonance at 13.23 GHz with a notch depth of 29.42 dB. The resonance of the fabricated test structures post-CNT in- tegration have varying resonances (16.43 GHz with a notch depth of 31.2 dB for one test structure and 13.58 GHz with a notch depth of 30.1 dB for the other). The variation is due to non-uniform CNT bridges across the transmission line. iv Dedicated to my parents, James and Beth Bane v ACKNOWLEDGMENTS I would like to express my gratitude to all the people whose support and encouragement has helped me complete my master’s thesis. First, I would like to thank my advisors Dr. Guru Sub- ramanyam and Dr. Karolyn M. Hansen. Throughout my time as an undergraduate and graduate student, Dr. Subramanyam has been advising me and has taught me much about microelectronics. When I met Dr. Hansen at the beginning of my time as a graduate student, she took me under her wing and taught me a great deal of biology. I am very grateful to both my advisors for their patience, expertise, and guidance. Additionally, I want to thank Dr. Banerjee for agreeing to be a part of my thesis committee. I am appreciative of the Dayton Area Graduate Studies Institute (DAGSI) Fellowship and the Graduate Student Summer Fellowship programs for providing funding for my thesis. I am very grateful to Dr. Elizabeth Downie, the director of DAGSI, and Dr. Joshua Hagen, my DAGSI sponsor, for giving me this opportunity. I would like to give a special thanks to Dr. Fahima Ouchen and Dr. James Grote at the Air Force Research Lab’s Material’s Directorate. Dr. Ouchen graciously opened her lab to me, helped in the fabrication of the test structures, and taught me much about biopolymers. I would like to thank Dr. Eunsung Shin for doing the metal deposition for the structures. I would like to thank Hailing Yue for teaching me how to perform measurements with the Vector Network Analyzer, teaching me how to use the Applied Wave Research Design Environment software, and answering any questions I had. I would like to thank Kuan-Chang Pan for his help. I would like to thank Kaushik Annam for guiding me in the carbon nanotube alignment. I would like to thank Dr. Jayne Robinson and Caitlin vi Bojanowski for granting me access to and helping me use the Epi-Fluorescent Microscope. Also, I would like to thank Dr. Charles Browning and Dr. Li Cao for allowing me to use the Kruss Drop Shape Analysis System 100. I would also like to thank my dogs Bella, Lotus, and Summer for our sessions of dog therapy. Lastly, I would like to thank my parents, James and Beth Bane, for all their love and support. Their constant encouragement and occasional prodding have driven me to complete my thesis. vii TABLE OF CONTENTS ABSTRACT........................................... iii DEDICATION.........................................v ACKNOWLEDGMENTS.................................... vi LIST OF FIGURES....................................... xi LIST OF TABLES....................................... xiii LIST OF ABBREVIATIONS AND NOTATIONS....................... xiv I. INTRODUCTION....................................1 1.1 Objectives.....................................2 1.2 Significance.....................................3 1.3 Outline.......................................4 II. BACKGROUND.....................................5 2.1 Capacitive Sensors.................................5 2.2 Resonant Sensors..................................6 2.3 Carbon Nanotubes (CNTs).............................7 2.4 Biopolymers....................................7 2.4.1 Silk.....................................8 2.4.2 Deoxyribonucleic Acid-Cetyltrimethylammonium Chloride (DNA-CTMA)8 2.4.3 Guanine..................................9 2.5 Proteins....................................... 10 2.5.1 Structure.................................. 10 2.5.2 Protein-Based Sensors........................... 12 2.6 Biomolecule Immobilization............................ 13 2.7 Model Analytes................................... 14 2.7.1 Orexin A.................................. 14 2.7.2 Trimethylamine.............................. 15 2.8 Summary...................................... 16 viii III. TEST STRUCTURES.................................. 18 3.1 Capacitive Test Structure (CTS).......................... 18 3.2 Resonant Test Structure (RTS)........................... 19 3.3 Electrical Model.................................. 21 3.4 RF Bridge...................................... 23 3.5 Half-Wavelength Resonator............................ 24 3.6 Summary...................................... 25 IV. EXPERIMENTAL PROCEDURE............................ 26 4.1 Test Structure Analysis............................... 26 4.2 Contact Angle Measurements........................... 27 4.3 Biotin-Streptavidin Model............................. 28 4.4 CNT Bridge Formation............................... 31 4.5 Summary...................................... 32 V. RESULTS AND DISCUSSION............................. 33 5.1 CTS Results.................................... 33 5.2 RTS Results.................................... 35 5.3 Contact Angle Measurement Results........................ 37 5.4 Biotin-Streptavidin Model Results......................... 37 5.5 RF Bridge Simulation............................... 40 5.6 Fabricated RF Bridge Test Structure Results.................... 42 5.7 Half-Wavelength Resonator Simulation...................... 45 5.8 Summary...................................... 46 VI. CONCLUSIONS AND FUTURE WORK........................ 47 6.1 Resonant Capacitive Sensor............................ 47 6.2 RF Bridge and Half-Wavelength Resonator.................... 50 BIBLIOGRAPHY........................................ 51 Appendices: A. CTS MEASUREMENTS................................. 57 B. RTS MEASUREMENTS................................. 58 ix C. CONTACT ANGLE MEASUREMENTS........................ 59 D. BUFFER AND CHEMICAL PROTOCOLS....................... 60 x
Details
-
File Typepdf
-
Upload Time-
-
Content LanguagesEnglish
-
Upload UserAnonymous/Not logged-in
-
File Pages79 Page
-
File Size-