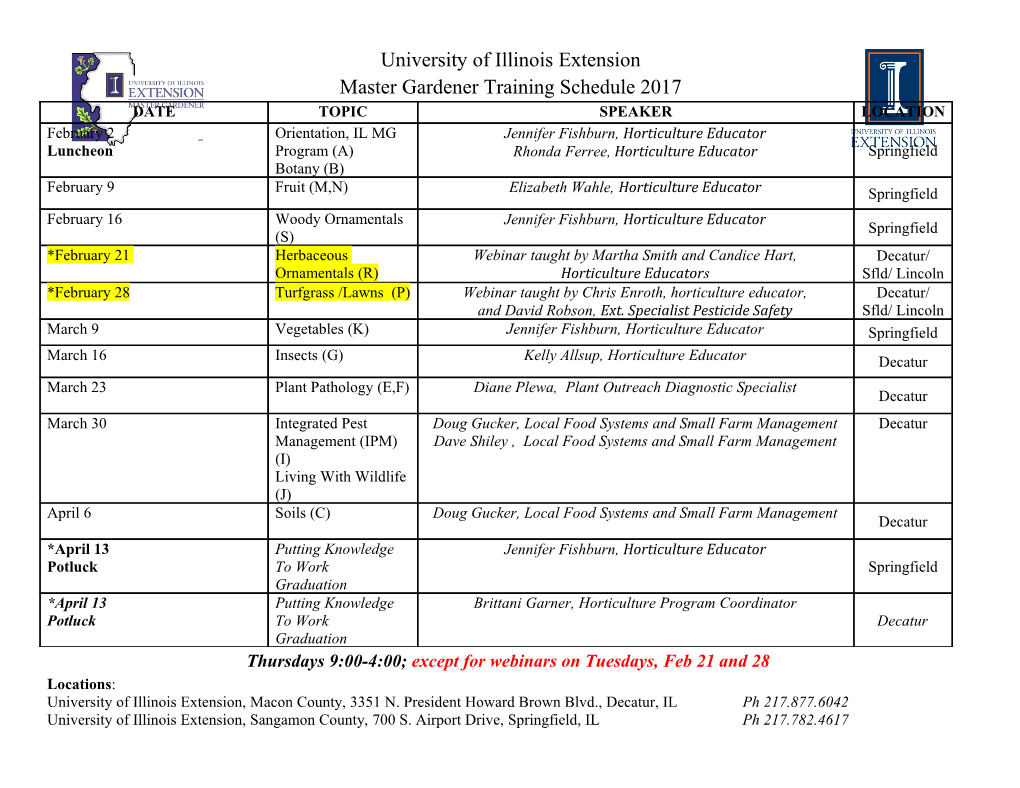
https://doi.org/10.1130/G48995.1 Manuscript received 26 February 2021 Revised manuscript received 15 April 2021 Manuscript accepted 20 April 2021 © 2021 The Authors. Gold Open Access: This paper is published under the terms of the CC-BY license. Explosive caldera-forming eruptions and debris-filled vents: Gargle dynamics Greg A. Valentine* and Meredith A. Cole Department of Geology, University at Buffalo, 126 Cooke Hall, Buffalo, New York 14260, USA ABSTRACT conservation equations solved for both gas and Large explosive volcanic eruptions are commonly associated with caldera subsidence and particles, which are coupled through momentum ignimbrites deposited by pyroclastic currents. Volumes and thicknesses of intracaldera and (drag) and heat exchange (as in Sweeney and outflow ignimbrites at 76 explosive calderas around the world indicate that subsidence is com- Valentine, 2017; Valentine and Sweeney, 2018). monly simultaneous with eruption, such that large proportions of the pyroclastic currents The same approach was used to study discrete are trapped within the developing basins. As a result, much of an eruption must penetrate phreatomagmatic explosions in debris-filled its own deposits, a process that also occurs in large, debris-filled vent structures even in the vents (Sweeney and Valentine, 2015; Sweeney absence of caldera formation and that has been termed “gargling eruption.” Numerical et al., 2018), but here we focus on sustained dis- modeling of the resulting dynamics shows that the interaction of preexisting deposits (fill) charges. The simplified two-dimensional (2-D), with an erupting (juvenile) mixture causes a dense sheath of fill material to be lifted along axisymmetric model domain extends to an alti- the margins of the erupting jet. This can cause an eruption that would otherwise produce a tude of 3 km (5640 m in one case) and radially buoyant plume and fallout deposits to instead form pyroclastic currents as the dense sheath away from the axis of symmetry to a horizontal drives pulsing jet behavior. Increasing thickness of fill amplifies the time variation in jet distance of 6 km (Fig. 2). The 2-D approach height. Increasing the fill grain size relative to that of the juvenile particles can result in a does not account for the three-dimensional (3-D) much higher jet due to poorer mixing between the dense sheath and the dilute jet core. In structure of eddies that govern entrainment; sim- all cases, material collapses along the entire height of the dense sheath rather than from the ulated jet heights and transitions from dense jet top of a simple fountain. These gargle dynamics provide strong backing for processes that to buoyant plume are therefore approximate but have been inferred to result in intraplinian ignimbrites and simultaneous deposition from are expected to be reasonable (e.g., Nourazar high- and low-energy pyroclastic currents. and Safavi, 2017). Ambient air in the domain has density and temperature determined initially by INTRODUCTION portions of the eruptions had to penetrate their the standard atmospheric profile (Sparks et al., Large-volume, explosive volcanic erup- own fresh deposits, a process referred to as “gar- 1997). The eruptive mixture of hot particles tions eject tens to thousands of cubic kilome- gling eruption” by Wilson and Hildreth (1997). and H2O vapor enters the domain from the bot- ters of magma. Calderas—subsidence features Eruptions that do not involve major caldera col- tom boundary adjacent to the symmetry axis formed by rapid evacuation of large volumes of lapse may also have very wide, debris-filled vent at a constant rate; these particles are referred magma—are ubiquitous features of the larger structures through which continued eruption to as juvenile particles. In all simulations, this eruptions. Studies of eroded calderas (e.g., Lip- must penetrate (e.g., 1912 CE Novarupta vent inflow boundary (vent) has a 100 m radius. man, 2000) and drill cores in young examples in Alaska, USA; Hildreth and Fierstein, 2012). The remainder of the bottom boundary has a (e.g., Nielson and Hulen, 1984) indicate that We present numerical modeling that explores no-slip condition while the top and right bound- poorly sorted, pumice-rich deposits of pyroclas- the effects of gargling on eruption dynamics. aries allow outflow. Caldera fill is represented tic currents (ignimbrites) within many calderas Although the simulations are greatly simplified by a bed of particles (porosity 40 vol%) that compose significant portions of the total vol- compared to natural cases, they provide strong initially extends from the symmetry axis to the umes erupted (Fig. 1A; Item S1 in the Supple- theoretical backing for processes that have been inner edge of a caldera rim; these preexisting mental Material1); the intracaldera deposits are inferred from field studies and that have major particles are referred to as fill particles. The rim normally significantly thicker than ignimbrites effects on explosive eruptions and their deposits. is simply represented as a rectangular obstacle that flowed out of the calderas from the same of a defined height, located between 1000 and eruptions (outflow; Fig. 1B). These data are MODELING APPROACH AND RESULTS 1500 m from the axis. Although natural calde- consistent with pyroclastic currents having been We model the dynamics of eruption through ras have more complex 3-D topography and can partially trapped within progressively deepen- particle layers with thicknesses between 50 and erupt from vents with a range of sizes, shapes, ing calderas during eruption and implies that 100 m that represent freshly deposited caldera- and distances from developing caldera margins fill ignimbrite or vent debris. Fluid flow is mod- and which can be active individually or simul- *E-mail: [email protected] eled with time-dependent, compressible-flow taneously, it is necessary to use our abstracted 1Supplemental Material. Item S1 (global caldera data), and Item S2 (modeling approach). Please visit https://doi .org/10.1130/GEOL.S.14772804 to access the supplemental material, and contact [email protected] with any questions. CITATION: Valentine. G.A., and Cole, M.A., 2021, Explosive caldera-forming eruptions and debris-filled vents: Gargle dynamics: Geology, v. 49, p. XXX–XXX, https://doi.org/10.1130/G48995.1 Geological Society of America | GEOLOGY | Volume XX | Number XX | www.gsapubs.org 1 Downloaded from http://pubs.geoscienceworld.org/gsa/geology/article-pdf/doi/10.1130/G48995.1/5346464/g48995.pdf by guest on 24 September 2021 AB Figure 1. Plots of intra- caldera and outflow ignimbrite volumes (A) and thicknesses (B) from a compilation of pub- lished data for 76 calderas around the world (see Item S1 [see footnote 1]). approach in order to gain a basic understand- of sheath material occurs continuously along ∼300 m/s as it emerges from the fill, maintains a ing of processes. Governing equations, mate- its height. This collapsing mixture, which is bulk particle density of 3–4 kg/m3 to a height of rial properties, information about the numerical dominated by recycled fill particles, feeds later- ∼1500 m before the coarser fill particles begin code, boundary conditions for simulations, and ally flowing pyroclastic currents Figs. 2B( and to contribute appreciably to its density. Above comparison of grid-size effects are detailed in 2C). Collapse of a dense outer sheath contrasts ∼1500 m, the bulk particle density is ∼5 kg/m3 Item S2. with a simple fountain structure that results up to a height of ∼3500 m, where the density To emphasize the important effects that cal- when a dense mixture erupts unimpeded into abruptly drops to values that are similar to or dera fill can have on eruption dynamics, we first air, where material collapses from the top of less than that of the surrounding ambient air focus on two simulations that have identical a fountain via a stem structure that impacts (∼0.35 kg/m3). This density drop corresponds conditions of the erupting mixture (Fig. 2). The the ground some distance from the jet (Fig. 2B to an abrupt transition from negative buoyancy first (Fig. 2A) is an eruption into air (no fill); inset; Valentine et al., 1992; Neri et al., 2003). below to positive buoyancy above (Fig. 3B). this erupting mixture forms a vertical jet at its A buoyant gas-particle mixture rises from the At the level of the transition, particles fall back base. In the absence of expansion or interac- top of the currents and along the outer part of toward the ground along the outer margin of the tion with surrounding air, this jet would lose its the complex jet structure. Thus, the presence dense sheath. The buoyancy transition maintains kinetic energy at an altitude of ∼500 m and col- of a relatively thin (50 m) intracaldera deposit a relatively constant height, fluctuating by only lapse to the ground to form a fountain structure can cause pyroclastic currents in an eruption a few hundred meters. Material from the dense and outgoing pyroclastic currents. However, in that would otherwise produce a buoyant plume sheath collapses along its outer margin during this case, which erupts with a relatively low and fallout deposits. the entire evolution of the jet and overlying density of ∼4 kg/m3 (corresponding to 4 wt% Increasing the thickness and grain size buoyant plume, feeding thin pyroclastic currents H2O and 0.2 vol% particles), the mixture attains of initial fill amplifies the effects described that are dominated by coarser fill particles. The an altitude of ∼700 m, where it spreads later- above, including the time-dependent behavior. finer juvenile component mainly falls from the ally (Fig. 2A). Interaction with the atmosphere For example, a simulation with the same erup- jet top, reaching the ground and increasingly reduces the mixture density so that instead of tive mixture as described above (adjusted to contributing to the currents at later times.
Details
-
File Typepdf
-
Upload Time-
-
Content LanguagesEnglish
-
Upload UserAnonymous/Not logged-in
-
File Pages5 Page
-
File Size-