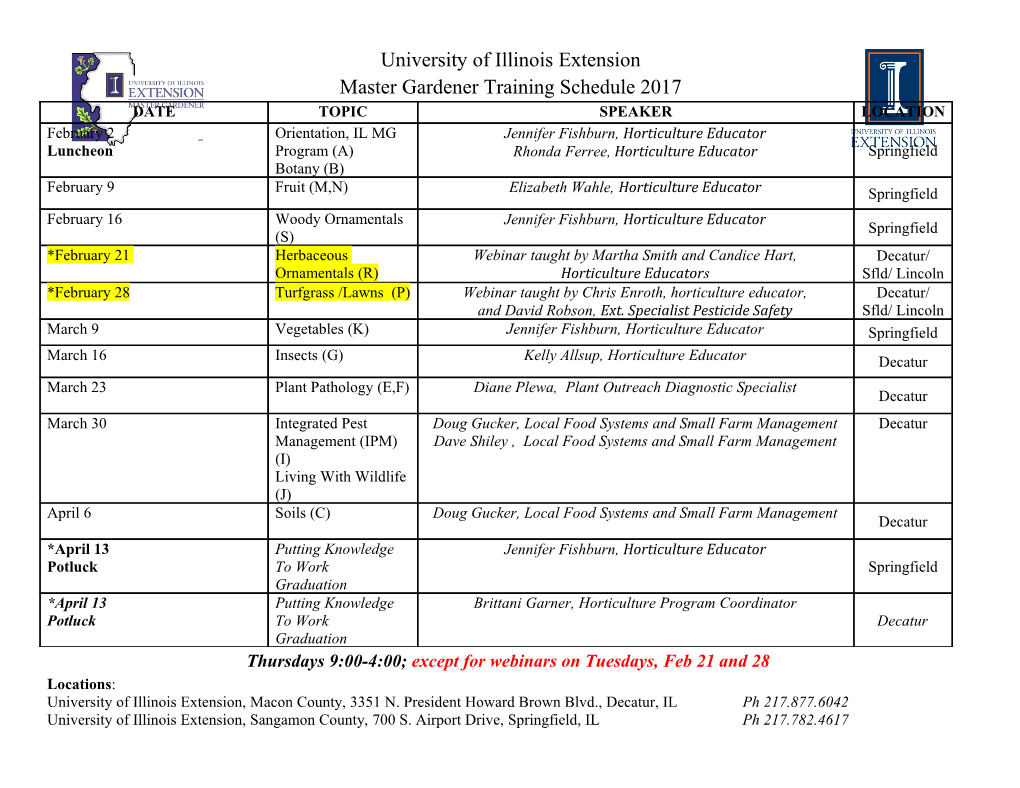
A Multi-Channel, Impedance-Matching, Wireless, Passive Recorder for Medical Applications (2019 Version) Dissertation Presented in Partial Fulfillment of the Requirements for the Degree Doctor of Philosophy in the Graduate School of The Ohio State University By Wei-Chuan Chen, B.Eng. Graduate Program in Electrical and Computer Engineering The Ohio State University 2019 Dissertation Committee: Asimina Kiourti, Co-Advisor John L. Volakis, Co-Advisor Liang Guo Daniel Rivers © Copyright by Wei-Chuan Chen 2019 Abstract This dissertation presents a new technology for batteryless and wireless neu- rorecording system which can be applied clinically. Two clinical issues of this type of neural implant are the 1) multichannel operation and 2) high impedance and DC voltage offset from the brain electrode impedance. To resolve these two problems, one wireless multichannel system and one brain electrode interface impedance-matching system are proposed respectively. To achieve multichannel operation, one photo- activated multiplexer is employed in the implant circuit. The interrogator additionally sends an infrared control signal for channel selection. Experimental results show that the proposed neuropotential recorder exhibits 20 µVpp sensitivity at all eight channels. The system is also in compliance with the strictest Federal Communications Com- mission standards for patient safety. Notably, the proposed approach is scalable to a much higher number of channels. On the other hand, to mitigate the high impedance and DC voltage offset of the brain-electrode interface, one self-biasing PNP Bipolar Junction Transistor (BJT) is adopted in the brain circuits. This self-biasing PNP BJT increases the overall system's impedance and maintains the system sensitivity while the high impedance is present. Measurement results demonstrate that emulated neuropotentials as low as 200 µVpp can be detected at a 33 kΩ electrode impedance. Together, these proposed techniques would lead the wireless neuro recorders to be applicable in real, in-vivo clinical applications. ii This is dedicated to my parents, my family, my friends and everyone ever helps me. iii Acknowledgments I would like to thank my advisors, Dr. Volakis and Dr. Kiourti, who have encour- aged and given their best support to me during the journey of my Ph.D. Its never easy to give guidance to the latest, state of art research topics. I am really indebted to them for their valuable advice on both academics and my personal development. I would like to thank my parents, who are always there for me and encourage me to pursue my dream, even though deep in their heart they know their son will not be able to be around them for a long time. I would like to thank my colleagues Cedric Lee, Brocks Delong, Md Asiful Islam (Asif), Jingni Zhong, Satheesh Bojja Venkatakrishnan, and Shubhendu Bhardwaj. It is an incredible experience to work alongside these talented people. I would like to thank my junior colleagues Jack Blauert, Vigyanshu Mishra, Saad Alharbhi, Katrina Guido, Keren Zhu, and Ramandeep Vilhkhu. I really enjoyed every event we shared and celebrated together in the ESL. Hope you guys have a good time in the lab and earn the degree smoothly. I would like to thank my friends who have ever helped me and accompanied me through the toughest time. I will not be able to accomplish my degree without your support. Finally, I would like to thank my significant other, I-Shan Tsai, for her love and patience. iv Vita May 12, 1992 . Born - Taipei, Taiwan 2014 . .B.Eng. 2015-present . .Graduate Research Associate, Electrical & Computer Engineering, The Ohio State University. Publications Research Publications W. Chen, K. Guido and A. Kiourti, "Passive Impedance Matching For Implanted Brain-Electrode Interfaces," in IEEE Journal of Electromagnetics, RF and Microwaves in Medicine and Biology (published). W. Chen, C. W. L. Lee, A. Kiourti and J. L. Volakis, "A Multi-Channel Passive Brain Implant for Wireless Neuropotential Monitoring," in IEEE Journal of Electro- magnetics, RF and Microwaves in Medicine and Biology, vol. 2, no. 4, pp. 262-269, Dec. 2018. W. Chen, B. Delong, R. Vilkhu, and A. Kiourti, "Enabling Batteryless Wearables and Implants," in 2018 Applied Computational Electromagnetics Society Journal, vol. 33, no. 10, pp. 11061108, Oct. 2018. W. Chen, B. DeLong, R. Vilkhu and A. Kiourti, "Towards batteryless wearables and implants," in 2018 International Applied Computational Electromagnetics Society Symposium (ACES), Denver, CO, 2018, pp. 1-2. W. Chen, A. Kiourti and J. L. Volakis, "A Passive Multi-Channel Brain Implant for Wireless Neuropotential Monitoring," 2018 IEEE International Symposium on v Antennas and Propagation & USNC/URSI National Radio Science Meeting, Boston, MA, 2018, pp. 1133-1134. Fields of Study Major Field: Electrical and Computer Engineering Studies in: Electromagnetics RF and Microwave Circuits Antennas vi Table of Contents Page Abstract . ii Dedication . iii Acknowledgments . iv Vita.........................................v List of Tables . .x List of Figures . xi 1. Introduction . .1 1.1 Motivation and Challenges of Wireless and Fully-Passive Neurosens- ing Systems . .1 1.2 Neural Signal Types and Deep Brain Sensing Applications . .2 1.2.1 Action Potential . .2 1.2.2 Field Potential . .3 1.3 State-of-the-Art Neural Implants . .5 1.3.1 Wired Neurosensors . .5 1.3.2 Wireless Neurosensor . .6 1.4 Former Single-Channel Wireless Fully-Passive Recorder . 20 1.5 Organization of this Thesis . 21 2. Wireless and Fully-Passive Neurosensing System Overview . 26 2.1 Block Diagram and Operation Principle . 26 2.2 Mixer Circuit . 27 2.3 Antenna Interface . 29 2.4 Interrogator System . 32 vii 3. Improved Interrogator System: Analysis and Design . 36 3.1 Phase Noise Analysis . 36 3.2 Self-Mixing and DC Offset . 38 3.3 Phase Noise Reduced Interrogator System . 39 4. Wireless and Fully-Passive Multi-Channel Neurorecorder . 41 4.1 Block Diagram and Operation Principle . 42 4.2 Antenna Interface . 45 4.3 Implanted Mixer . 48 4.4 Infrared Transceiver and Receiver Design . 49 4.5 System Performance . 51 4.5.1 Fabricated Prototype . 51 4.5.2 Measurement Setup . 51 4.5.3 Minimum detectable signal . 53 4.5.4 Specific Absorption Rate (SAR) . 55 5. Wireless and Fully-Passive Implant Match to High-Impedance Electrodes 57 5.1 Introduction to Electrodes and Detection Mechanism . 57 5.2 Modeling and Measurement of Electrode Impedance and DC offset voltage . 60 5.3 Block Diagram and Operation Principle . 63 5.4 System Performance . 66 5.4.1 Measurement Setup . 66 5.4.2 Stand-Alone Circuit Performance . 67 5.4.3 Integrated System Performance . 68 5.4.4 DC Offset Tolerance . 71 6. Conclusion . 74 6.1 Summary . 74 6.2 Key Contributions . 75 6.3 Future Work . 76 6.3.1 In-V ivo Measurement and Result . 76 6.3.2 In − vivo Measurements in Rats . 78 6.3.3 In − vivo Measurements in Human Subjects . 79 6.3.4 Recorded Signal Processing . 80 6.3.5 Channel Scalability . 81 6.3.6 System Miniaturization . 82 6.3.7 Antenna Misalignment and Choice . 83 viii Appendices 86 A. MATLAB Code for Genetic Algorithm . 86 A.1 Main Code . 86 A.2 Script Writing . 96 A.3 Restraint Check . 97 A.4 Simulation . 100 Bibliography . 103 ix List of Tables Table Page 1.1 Voltage And Frequency Range Of Signals Generated By The Human Brain . .5 2.1 Gain and Noise Figure of Components Applied in the Interrogator . 34 4.1 Comparison Between Proposed vs. Previously Wireless Implanted De- vices Without Integrated Circuits . 46 5.1 Node Voltage and BJT Operation Regions . 65 x List of Figures Figure Page 1.1 Typical action potential of a neuron [1]. .2 1.2 Integrated Neural Interface system block diagram [2]. .6 1.3 The neural recording microsystem: (a) illustration of the overall sys- tem; (b) simplified block diagram of the implanted microsystem dia- gram [3]. .8 1.4 Functional block diagram of the NPU-32 [3]. .9 1.5 Schematics of the dual panel brain implantable microsystem featuring an active brain sensor (microelectrode array integrated to amplifier IC) in the cortical unit, and hybrid A/D, control, and RF-IR(Infrared) telemetry in the cranial unit [4]. 10 1.6 (a) Photographic images showing an implantable 16-channel microsys- tem with a dual-panel liquid crystal polymer substrate. A spiral pat- tern of RF power receiving coil is clearly visible in the backside image. (b) Block diagram of the dual-panel microsystem showing neural sig- nal and power/clock flows among various micro- and optoelectronic components [4]. 11 1.7 Design overview of HermesC [5]. 12 1.8 HermesC System (A) Setup with animal in a metal home cage and receiving antenna on plastic cage window (B) Aluminum enclosure with stub antenna in lid (C) PCB with INI chip (D) Example video data [5]. 13 xi 1.9 Block diagram of Bluetooth-based single-unit recording system. The wireless data acquisition module is carried by the rat in a backpack that transmits data to a PC-based control subsystem. Microdrive wire electrodes were implanted in the CA1 of the hippocampus for sensing single-unit activities which were first amplified by the analog-front-end amplifier and then digitized by the ADC that connects to the MCU for Bluetooth wireless transmission to the host PC [6]. 14 1.10 Block diagram of the neural data acquisition system [7]. 15 1.11 Functional block diagram of INI3 microchip [8]. 16 1.12 Block diagram of NeuralWISP [9]. 17 1.13 Exploded model view of the hermetically packaged 100-ch fully im- plantable wireless neurosensing device [10]. 18 1.14 Circuit block diagram [10]. 19 1.15 Schematic of a general BMI system architecture. Such systems require multichannel sensors for neural recording, specialized IC for signal pro- cessing, programs for brain simulation, a wireless link to communicate with external unit, and finally a real-time brain mapping to effectively track and decode the neural activities.
Details
-
File Typepdf
-
Upload Time-
-
Content LanguagesEnglish
-
Upload UserAnonymous/Not logged-in
-
File Pages124 Page
-
File Size-