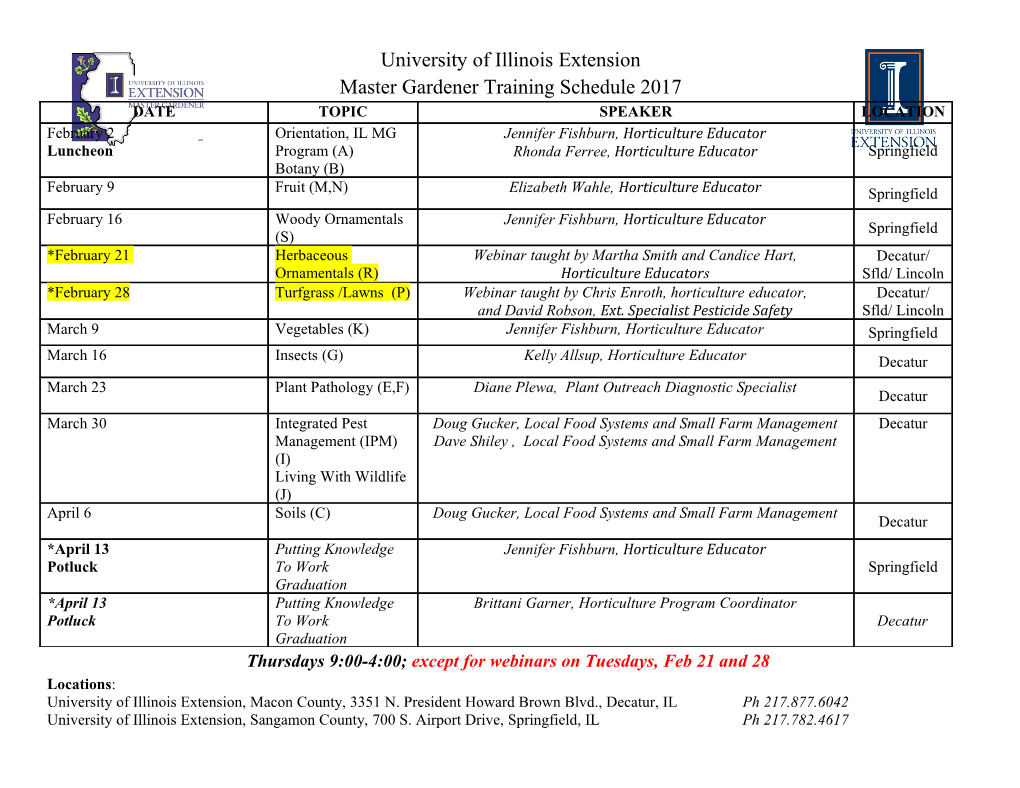
LIMNOLOGY and Limnol. Oceanogr. 61, 2016, 1984–2002 VC 2016 The Authors Limnology and Oceanography published by Wiley Periodicals, Inc. OCEANOGRAPHY on behalf of Association for the Sciences of Limnology and Oceanography doi: 10.1002/lno.10349 Long-term alkalinity trends in the Baltic Sea and their implications for CO2-induced acidification Jens Daniel Muller,*€ Bernd Schneider, Gregor Rehder Department of Marine Chemistry, Leibniz Institute for Baltic Sea Research, Warnemunde,€ Germany Abstract Anthropogenic CO2 emissions currently decrease open ocean pH, but on multi-millennial time scales intensified continental weathering is expected to contribute to increasing oceanic alkalinity (AT) and thus mitigate the acidification signal. The Baltic Sea is an ideal study site for such AT dynamics, due to its direct link to terrestrial processes, short water residence time and long history of AT measurements dating back to th the early 20 century. We compiled an extensive AT data set that revealed the highest data quality and cov- erage for the past two decades. Within that period, surface water AT levels increased throughout the Baltic Sea. The rates of change were highest in the low-saline, northern areas and decreased gradually toward con- 21 21 stant levels in the North Sea. The AT increase observed in the Central Baltic Sea (13.4 mmol kg yr ) and 21 21 the Gulf of Bothnia (17 mmol kg yr ) has compensated CO2-induced acidification by almost 50% and 100%, respectively. Further, the AT trends enhanced the CO2 storage capacity and stabilized the CaCO3 satu- ration state of the Baltic Sea over the past two decades. We discuss the attribution of the AT trends to poten- tial changes in precipitation patterns, continental weathering driven by acidic rain and increasing atmospheric CO2, agricultural liming and internal AT sources. Introduction water is formed, e.g. in the high latitudes of the North Alkalinity: global relevance and characteristics Atlantic (Lee et al. 2003). When the fossil fuel derived CO2 will invade the entire ocean interior on millennial time Total alkalinity (AT) is a measure of the acid-binding capacity of seawater. As one of four measureable parameters scales, the share of the oceanic uptake will increase to around 80% (Archer and Brovkin 2008). The oceanic uptake of the marine CO2 system, AT has gained increasing atten- tion within the field of climate change research. The impor- of CO2 is beneficial, because it counteracts the rising atmos- pheric CO2 levels and thus mitigates global warming. How- tance of AT for the carbon cycle can be exemplified by its ever, it comes at the expense of decreasing seawater pH. This control over the CO2 uptake and the pH of seawater. Cur- rently, the oceans absorb around 30% of the anthropo- process, termed ocean acidification (Doney et al. 2009), is expected to have global and potentially detrimental impacts genic CO2 released into the atmosphere (Khatiwala et al. 2013; Le Quer e et al. 2015), mainly due to equilibration on marine ecosystems (Hofmann et al. 2010; Kroeker et al. between the atmosphere and the surface ocean mixed layer. 2010). When CO2 dissolves in water, carbonic acid is formed and protons are released. The alkalinity of seawater deter- The penetration of anthropogenic CO2 into the ocean inte- mines the share of these protons that are neutralized and rior is controlled by the ocean circulation and today the thereby controls the CO uptake and the degree of acidifica- ongoing process can be observed at locations where deep 2 tion. For a given increase in atmospheric pCO2, more anthropogenic CO2 can be stored in seawater with higher AT. Although more CO2 could be taken up by high-AT sea- water, the degree of acidification would be smaller than at *Correspondence: [email protected] lower AT. Knowledge about spatio-temporal AT dynamics is Additional Supporting Information may be found in the online version of this thus crucial for a comprehensive understanding of the global article. carbon cycle and the impact of anthropogenic CO2 emis- sions on the marine CO system. This is an open access article under the terms of the Creative Commons 2 Attribution License, which permits use, distribution and reproduction in The exact definition of AT, as the excess of proton accept- any medium, provided the original work is properly cited. ors over proton donors (Dickson et al. 2007), is expressed by: 1984 Muller€ et al. Baltic Sea alkalinity trends ÂÃÂÃÂÃ ÂÃ A 5 HCO2 12 CO22 1 BðOHÞ2 1½OH2 1 HPO22 stored in the ocean to 90% (Archer and Brovkin 2008). How- T ÂÃ3 ÂÃ3 4 ÂÃ4 12 PO32 1 SiOðÞ OH 2 1½NH 1½HS2 2 H1 2½HF (1) ever, it may not dampen ocean acidification occurring on ÂÃ4 3 3 F 2 shorter, centennial timescales. 2 HSO4 2½H3PO4 1½minor bases2minor acids In contrast to the subtropical open ocean, AT –S rela- tions in marginal seas are much more variable because where [H1] is the free concentration of hydrogen ions. This F processes like riverine alkalinity input, CaCO formation definition implies that A is a conservative quantity with 3 T and denitrification (Cai et al. 2010; Gustafsson et al. 2014; respect to changes in temperature and pressure, although Jiang et al. 2014) superimpose on evaporation and precipi- the share of the various alkalinity components can vary. As tation effects. The dominant effect of riverine A input in a conservative quantity, A behaves exactly like salinity (S) T T marginal seas is typically reflected in linear A –S when two water parcels are mixed (Wolf-Gladrow et al. T relationships: 2007). This is not the case for non-conservative parameters of the marine CO2 system, like pCO2 and pH, which do not AT 5AT;01a3S (2) necessarily obey linear mixing relationships. Further, AT is not affected by the release or dissolution of CO2, because the where AT,0 corresponds to the river end-member alkalinity at 1 AT loss caused by the release of protons (H ) from the disso- S 5 0, and a is the slope representing the alkalinity change per ciation of carbonic acid (H2CO3) is balanced by the gain salinity unit. The direct link of coastal alkalinities to the river- 2 22 from produced bicarbonate (HCO3 ) and carbonate (CO3 ) ine AT input (which is in turn often impacted by anthropo- ions. Among the components that contribute to AT accord- genic activities) and the shorter residence time of alkalinity in 2 ing to Eq. 1, the major share can be attributed to HCO3 and coastal environments (which is basically equal to the water 22 CO3 that originate not from the AT-neutral dissolution of residence time) suggest that temporal changes in AT–S rela- CO2, but from riverine input of continentally weathered car- tionships may occur on timescales similar to that of CO2- bonate and silicate minerals. On oceanic scales this input is induced ocean acidification. A more comprehensive under- currently balanced by CaCO3 formation, sedimentation and standing of alkalinity dynamics in such systems is thus essen- burial as ultimate sinks (Sarmiento and Gruber 2006). tial to extent climate change research into the coastal zone. Zooming in from oceanic to coastal systems Focus on the Baltic Sea The open ocean surface AT is surprisingly stable, which The semi-enclosed Baltic Sea can be considered as one of reflects the long water residence time of 105 yr (Sundquist the world’s largest estuaries. The brackish water system is an 1991; Sarmiento and Gruber 2006). Most of the variation in ideal study site for the investigation of alkalinity dynamics, surface alkalinity can be attributed to precipitation and evap- due to the long history of biogeochemical studies including oration patterns (Millero et al. 1998; Jiang et al. 2014; Fry decades of alkalinity and salinity monitoring, a diverse, but et al. 2015). Because AT and salinity are affected similarly by well-defined bedrock structure in different parts of its large these processes, the salinity normalized alkalinity (nAT 5 AT drainage basin and a variety of existing biogeochemical 3 35/S) shows remarkably little spatial variability in the models. For this study we divide the Baltic Sea into five sub- subtropical gyres of the major ocean basins, e.g., 2291 6 4 areas, which accounts for the patterns of the surface AT–S mmol kg21 (mean 6 SD) between 30 8S and 30 8N in the distribution (Beldowski et al. 2010). The geographical bor- Atlantic (Millero et al. 1998; Friis et al. 2003). Likewise, the ders of the five subareas, which are the narrow Belt Sea and surface nAT in the Northern Atlantic did not exhibit signifi- Kattegat region (Kat), Central Baltic Sea (Cen), Gulf of Both- cant temporal changes over the past three decades (Bates nia (Bot), Gulf of Finland (Fin) and Gulf of Riga (Rig), are et al. 2012). Based on this constant AT background in the displayed in Fig. 1. The hydrography of the Baltic Sea is open ocean, acidification trends around 0.002 pH units yr21 characterized by inflowing, high saline water from the North can be predicted from future pCO2 scenarios “without major Sea that enters the deep basins of the Baltic Sea and gener- uncertainties” (Doney et al. 2009). These predictions match ates a permanent halocline. The outflow of low-saline sur- well with the currently observed pH trends at seven globally face waters, caused by river-runoff and positive net distributed time-series stations (Bates et al. 2014). precipitation, slightly exceeds the inflow. The mean salinity However, on longer time scales the oceanic alkalinity is of the Baltic Sea shows decadal, large-scale variations of up expected to increase. On millennial time scales (103 yr) to 1 salinity unit, but no long-term trend was found during anthropogenic CO2 will acidify the ocean interior and thus the past century (Winsor et al.
Details
-
File Typepdf
-
Upload Time-
-
Content LanguagesEnglish
-
Upload UserAnonymous/Not logged-in
-
File Pages19 Page
-
File Size-