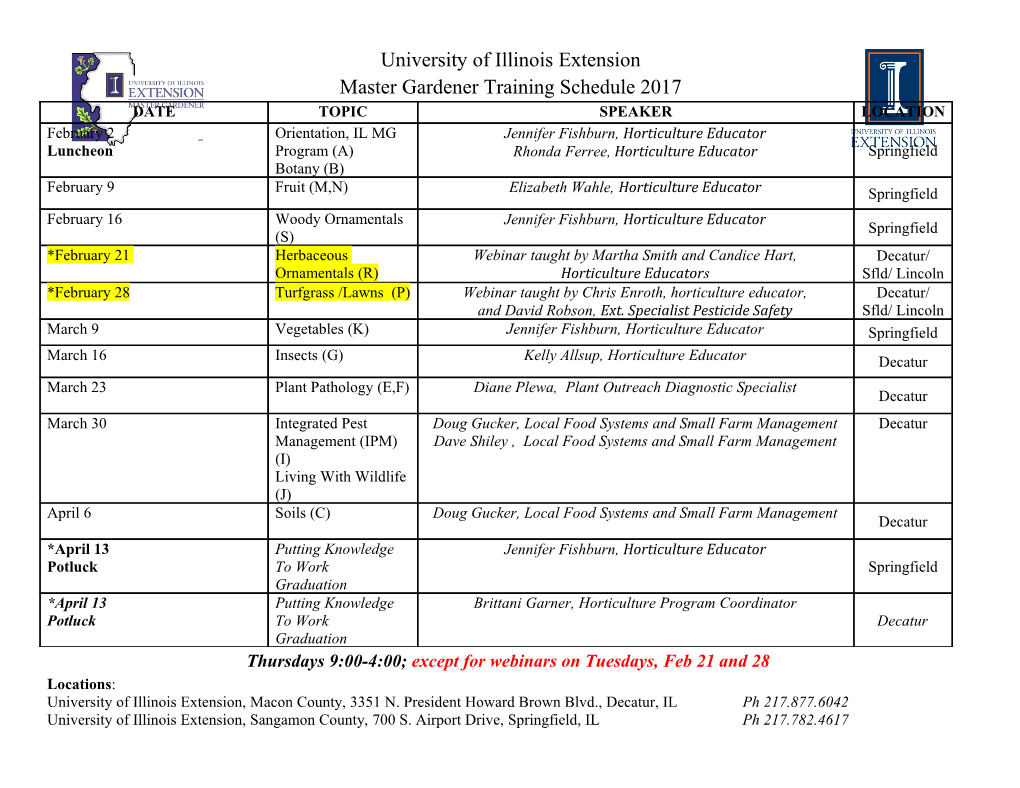
Georgia Southern University Digital Commons@Georgia Southern Manufacturing Engineering, Department of - Faculty Research and Publications Manufacturing Engineering, Department of 9-15-2020 Design of a Methanol Reformer for on-board Production of Hydrogen as Fuel for a 3kW High-temperature Proton Exchange Membrane Fuel Cell Power System Vladimir Gurau Georgia Southern University, [email protected] Adedayo Ogunleke Georgia Southern University, [email protected] F. Strickland Follow this and additional works at: https://digitalcommons.georgiasouthern.edu/manufact-eng-facpubs Part of the Manufacturing Commons Recommended Citation Gurau, Vladimir, Adedayo Ogunleke, F. Strickland. 2020. "Design of a Methanol Reformer for on-board Production of Hydrogen as Fuel for a 3kW High-temperature Proton Exchange Membrane Fuel Cell Power System." International Journal of Hydrogen Energy, 45 (56): 31745-31759: Elsevier. doi: https://doi.org/ 10.1016/j.ijhydene.2020.08.179 source: https://www.sciencedirect.com/science/article/pii/ S0360319920332067?via%3Dihub#! https://digitalcommons.georgiasouthern.edu/manufact-eng-facpubs/104 This article is brought to you for free and open access by the Manufacturing Engineering, Department of at Digital Commons@Georgia Southern. It has been accepted for inclusion in Manufacturing Engineering, Department of - Faculty Research and Publications by an authorized administrator of Digital Commons@Georgia Southern. For more information, please contact [email protected]. international journal of hydrogen energy 45 (2020) 31745e31759 Available online at www.sciencedirect.com ScienceDirect journal homepage: www.elsevier.com/locate/he Design of a methanol reformer for on-board production of hydrogen as fuel for a 3 kW High- Temperature Proton Exchange Membrane Fuel Cell power system * V. Gurau a, , A. Ogunleke b, F. Strickland a a Georgia Southern University, Department of Manufacturing Engineering, Statesboro, GA, 30458, USA b Georgia Southern University, Mechanical Engineering Department, Statesboro, GA, 30458, USA highlights graphical abstract CFD is an essential tool for deter- mining process parameters of methanol reformers. Operating conditions of MEAs are critical constraints in methanol reformer design. Reformer design must result in high conversion efficiency and small reformer volume. article info abstract Article history: The method of Computational Fluid Dynamics is used to predict the process parameters Received 31 January 2020 and select the optimum operating regime of a methanol reformer for on-board production Received in revised form of hydrogen as fuel for a 3 kW High-Temperature Proton Exchange Membrane Fuel Cell 28 May 2020 power system. The analysis uses a three reactions kinetics model for methanol steam Accepted 21 August 2020 reforming, water gas shift and methanol decomposition reactions on Cu/ZnO/Al2O3 cata- Available online 15 September 2020 lyst. Numerical simulations are performed at single channel level for a range of reformer operating temperatures and values of the molar flow rate of methanol per weight of Keywords: catalyst at the reformer inlet. Two operating regimes of the fuel processor are selected Methanol reformer which offer high methanol conversion rate and high hydrogen production while simulta- High-temperature proton exchange neously result in a small reformer size and a reformate gas composition that can be membrane fuel cell tolerated by phosphoric acid-doped high temperature membrane electrode assemblies for Methanol steam reforming proton exchange membrane fuel cells. Based on the results of the numerical simulations, Fuel cell system optimization the reactor is sized, and its design is optimized. Computational fluid dynamics © 2020 The Author(s). Published by Elsevier Ltd on behalf of Hydrogen Energy Publications LLC. This is an open access article under the CC BY-NC-ND license (http:// creativecommons.org/licenses/by-nc-nd/4.0/). * Corresponding author. E-mail address: [email protected] (V. Gurau). https://doi.org/10.1016/j.ijhydene.2020.08.179 0360-3199/© 2020 The Author(s). Published by Elsevier Ltd on behalf of Hydrogen Energy Publications LLC. This is an open access article under the CC BY-NC- ND license (http://creativecommons.org/licenses/by-nc-nd/4.0/). 31746 international journal of hydrogen energy 45 (2020) 31745e31759 3 Nomenclature Vcat Volume of the active catalyst bed ðm Þ V/cell Voltage delivered by a single cell in the fuel cell A Active area of the fuel cell MEA (cm2) : Â stack (V) aref Specific area density of the reformer catalyst (3 5 W=F ; Inverse molar flow rate of methanol at inlet per 108m2=m3) CH3 OH in weight of catalyst (kg s=mol) cp Specific heat capacity at constant pressure (J=kg K) ÀX xi Molar fraction of component i C1 À C7 Adsorption coefficients of surface spices (bar ) y Mass fraction of component i T; T i CS1 CS1A Total surface concentration of active sites for MSR and WGS reactions (mol=m2) Greek symbols T; T ε CS2 CS2A Total surface concentration of active sites for MD V Volume porosity of the catalyst bed (0.36) reaction (mol=m2) ε Area porosity of the catalyst bed (0.36) 2= Di Diffusion coefficient of species i (m s) z Methanol conversion rate F Faraday constant (96,485 C/eq) h Reformer thermal efficiency (%) h Specific enthalpy (J=kg) l Thermal conductivity (W=mK) 2 l i Fuel cell current density (A/cm ) i Stoichiometric ratio of component i in À9 2 KV Permeability of catalyst bed (1:0 Â 10 m ) electrochemical reaction (1.2 for hydrogen, 2.0 for Ki Equilibrium constant for reaction i air) 2= ki Rate constant of reaction i (m mol s) m Dynamic viscosity (kg=ms) ð = Þ u_ = 3 LHV Lower heating value MJ kg i Source term for species i (g m s) = 3 Mi Molecular weight of species i (kg kmols) r Density (kg=m ) _ = mi Mass flow rate of species i (kg s) F Relative humidity of the reformate gas (%) n Number of cells in the fuel cell stack FW Parameter relating the gas composition to the _ = ni Molar flow rate of species i (kmol s) WGS equilibrium p Pressure (Pa) Subscripts pi Partial pressure of species i (bar) MD Methanol decomposition reaction Pe Electrical power provided by the fuel cell stack MSR Methanol steam reforming reaction (3000 W) _ WGS Water gas shift reaction Q Source term for energy equation (W=m3) in Property at reformer inlet q_ Wall heat flux (W=m2) out Property at reformer outlet R Universal gas constant (8314 J=kmol K) _ = 2 eq Equivalent property rj Rate of reaction j (mol m s) mix Property of the gas mixture S/M Steam to methanol molar ratio at reformer inlet cat Catalyst T Absolute temperature (K) U Velocity vector (m/s) monoxide concentrations up to 3% [4], compared to less than Introduction 100 ppm in low-temperature PEMFCs. These benefits make HT- PEMFCs particularly suitable for reformate gas-operating auto- There is a need to demonstrate power systems with a high fuel- motive systems with a simplified design, in which the prefer- to-electricity conversion efficiency used to extend the endur- ential oxidation stage in the fuel processing line can be ance of autonomous terrestrial vehicles and unmanned aerial eliminated. Also, they result in fuel cell power systems with a e vehicles (UAV) [1 3]. Fuel cells are energy conversion devices significant reduction in cost and complexity resulting from a that convert the chemical energy of hydrogen directly to elec- smaller radiator in the cooling loop and the elimination of the tricity at higher conversion efficiencies than other systems. humidifiers in the gas feed loops. In addition to these advan- Proton Exchange Membrane Fuel Cells (PEMFCs) deliver high- tages, HT-PEMFCs offer the ability to use the fuel cell stack waste power density and offer the advantages of low weight and vol- heat to boil water or heat space when used as a combined heat ume, rapid start-up, and better durability compared with other and power (CHP) system, increasing thus the system efficiency fuel cells, features that make them particularly suitable for substantially when compared to conventional low temperature automotive, underwater and aerial applications. High- fuel cells power systems. Temperature PEMFCs (HT-PEMFCs) are capable of operating Even though hydrogen gas has the highest heat of com- between 120 C and 180 C without external humidification, bustion (MJ/kg), its energy density (MJ/m3) is lower compared which renders them significant benefits over the low- to other fuels. Despite having a higher energy conversion ef- temperature PEMFCs. These benefits include simplified water ficiency than other systems, a long-endurance automotive and thermal management, faster electrode kinetics for both fuel cell power system using compressed hydrogen gas would electrode reactions, and an improved anode tolerance to carbon international journal of hydrogen energy 45 (2020) 31745e31759 31747 result in a system having a substantial volume due to the size It can be noted that only two of these reactions are linearly of the gas cylinders. However, methanol is an energy carrier independent and any one of them can be expressed as the with energy density seven times higher than that of com- algebraic sum of the other two. Because of this, there has been pressed hydrogen gas available using today’s technology. disagreement in the past regarding the reactions that must be Methanol steam reformers convert methanol solution into a included in a kinetic model of the process of methanol steam hydrogen rich gas that contains as well carbon monoxide, reforming on Cu/ZnO/Al2O3 catalyst. Previous numerical carbon dioxide and traces of unused water and methanol models have used one or two of the three possible reactions vapors in a catalytic reaction at temperatures as low as [7,8,13e17] and have considered that the other reactions were 200 Ce350 C and using inexpensive copper-based catalysts either at equilibrium, or that their reaction rates were negli- [5].
Details
-
File Typepdf
-
Upload Time-
-
Content LanguagesEnglish
-
Upload UserAnonymous/Not logged-in
-
File Pages16 Page
-
File Size-