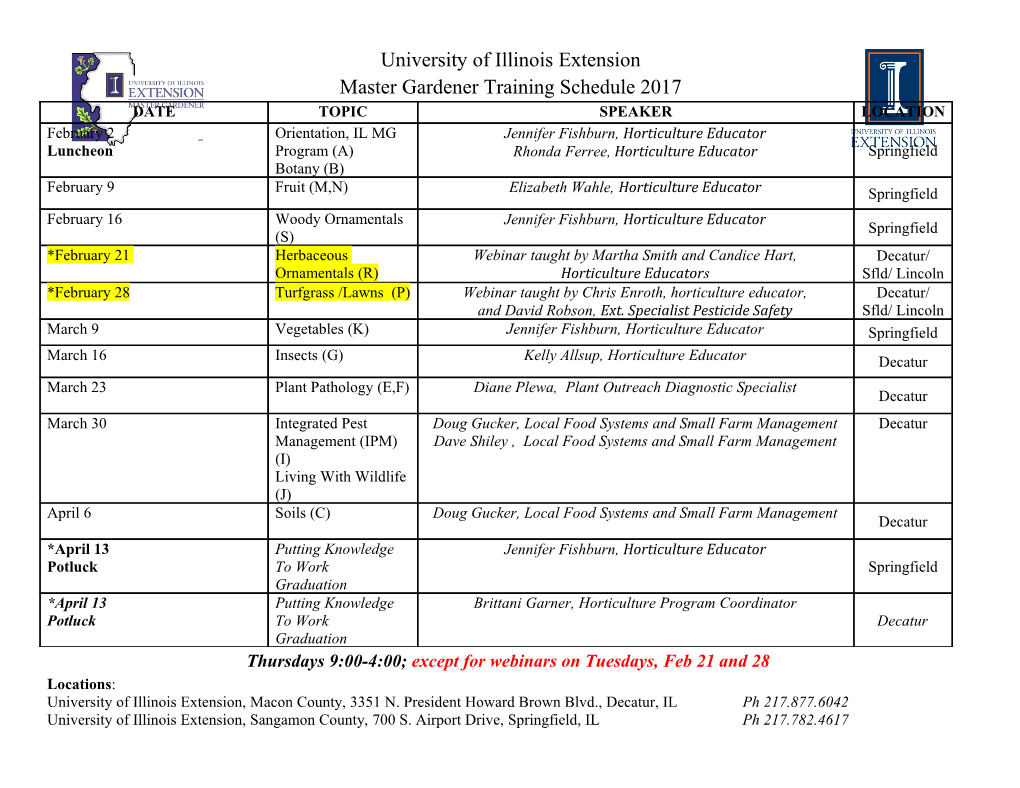
BIOMOLECULES (INTRODUCTION, STRUCTURE AND FUNCTIONS) Nucleic acids Smita Rastogi 1 & U. N. Dwivedi 2 1 Lecturer, Department of Biotechnology, Integral University, Lucknow, India 2 Professor, Department of Biochemistry, University of Lucknow, Lucknow-226007, India 6-Jun-2006 (Revised 12-Jun-2007) CONTENTS Composition of nucleic acids Generalized structural units of nucleic acids Nucleosides Nucleotides or Nucleoside 5’-triphosphates Oligonucleotides Nomenclature of nucleic acids Structural levels of nucleic acids Deoxyribonucleic acid (DNA) Ribonucleic acid (RNA) Structure Types of RNA Messenger RNA (mRNA) Ribosomal RNA (rRNA) Transfer RNA (tRNA) Heterogeneous nuclear RNA (hnRNA) Key words Deoxyribose sugar, DNA, mRNA, Purines, Pyrimidines, Ribose sugar, rRNA, tRNA The nucleic acids are the molecular repositories for genetic information and referred to as the ‘Molecules of Heredity’. Although the name nucleic acid suggests their location in the nuclei of cells, yet some of them are, however, also present in the cytoplasm. The nucleic acids are the hereditary determinants of living organisms. They are the macromolecules present in most living cells either in the free state or bound to proteins as nucleoproteins. There are two types of nucleic acids, deoxyribonucleic acid (DNA) and ribonucleic acid (RNA). Both are present in all plants and animals. Viruses also contain nucleic acids, however, unlike a plant or animal has either RNA or DNA, but not both. DNA is found mainly as a component of chromatin material of the cell nucleus whereas most of the RNA (90%) is present in the cell cytoplasm and the remaining (10%) in the nucleolus. Extranuclear DNA also exists, for e.g., in mitochondria and chloroplasts. Composition of nucleic acids Nucleic acids are biopolymers of high molecular weight with mononucleotide as their repeating units. Each mononucleotide consists of the following: (A) Nitrogenous bases (B) Phosphoric acid (C) Pentose sugars (A) Nitrogenous bases Two types of major nitrogenous bases, which account for the base composition of DNA or RNA, are found in all nucleic acids. These are: a) Purine bases b) Pyrimidine bases The purine and pyrimidine bases found in nucleic acids are listed in Table 1 and their structures are given in Fig. 1. Table 1: Purine and pyrimidine bases in DNA and RNA Name of Purine or Molecular Molecular Properties Found in base pyrimidine formula weight (Da) DNA and / or RNA Adenine Purine C5H5N5 135.15 White, crystalline DNA and (A) RNA Guanine Purine C5H5ON5 151.15 Colourless, DNA and (G) crystalline RNA Cytosine Pyrimidine C4H5ON3 111.12 White, crystalline, RNA and (C) first isolated from DNA Guano (bird manure) Thymine Pyrimidine C5H6O2N2 126.13 White, crystalline, DNA only (T) first isolated from thymus tissue Uracil (U) Pyrimidine C4H4O2N2 112.10 White, crystalline RNA only 2 Nitrogenous bases Pyrimidines H H O N H CH3 N N O N O N H H Thymine Cytosine In RNA, Uracil is present instead of Thymine O H N O N H Uracil H H N N O N H N N N N H N N N H H H Adenine Guanine Fig. 1: Structure of purine and pyrimidine bases Both the purine and pyrimidine bases are planar molecules, owing to their π-electron clouds. Purine and pyrimidine bases are hydrophobic and relatively insoluble in water at the near neutral pH of cell. Purines can exist in syn or anti forms; pyrimidines can exist in anti form because of steric interference between the sugar and carbonyl oxygen at C-2 of pyrimidine. Besides, the major nitrogenous bases, some minor bases also called modified nitrogenous bases (purines and pyrimidines) also occur in polynucleotide structures. Some naturally occurring forms of modified purines are hypoxanthine, xanthine, uric acid, 6-methyladenine (6-Me), 6-dimethyladenine (6-DiMe), 6-N-isopentenyladenine (6-IPA), 1- 3 methylguanine (1-MeG), 2-dimethylguanine (2-DiMeG). Among the modified purines, some are found in tRNA (described later). Methylation is the most common form of purine modification in microorganisms. The presence of such methylated purines is also suggested in plant genomes. Some naturally occurring forms of modified pyrimidines (e.g. 5,6-dihydrouracil, pseudouracil, 4-Thiouracil etc.) are common in tRNA (described later). Other examples include 5-methylcytosine (5-MeC) and 5-hydroxymethylcytosine. The 5-methylcytosine is a common component of higher plant and animal DNA. Infact up to 25% of the cytosine residues of plant genome are methylated. The DNA of plants is richer in 5-MeC than the DNA of animals. The DNA of the T-even bacteriophages (T2, T4) of E. coli has no cytosine but instead has 5-hydroxymethylcytosine and its glucoside derivatives. (B) Phosphorus Phosphorus, present in the backbone of nucleic acids, is a constituent of phosphodiester bond that links the two sugar moieties. The molecular formula of phosphoric acid is H3PO4. It contains three monovalent hydroxyl groups and a divalent oxygen atom, all linked to the pentavalent phosphorus atom. (C) Sugar Both DNA and RNA contain five-carbon ketose sugar, i.e. a pentose sugar. The essential difference between DNA and RNA is the type of sugar they contain. RNA contains the sugar D-ribose (hence called ribonucleic acid, RNA) whereas DNA contains its derivatives 2’-deoxy-D-ribose, where the 2’-hydroxyl group of ribose is replaced by hydrogen (hence called deoxyribonucleic acid, DNA). Sugars are always in closed ring β-furanose form in nucleic acids and hence are called furanose sugars because of their similarity to the heterocyclic compound furan. The structure of pentose sugars present in DNA and RNA are shown in Fig. 2. Sugars 5' 5' OH CH OH OH CH OH 2 O 2 O HC 4' 1' CH HC 4' 1' CH 3' 2' 3' 2' HC CH HC CH OH OH OH H D-Ribose 2'-Deoxyribose Fig. 2: Structure of sugars present in nucleic acids 4 The structural difference in the sugars of DNA and RNA, though minor, confers very different chemical and physical properties upon DNA than RNA. RNA is much stiffer due to steric hindrance and more susceptible to hydrolysis in alkaline conditions, perhaps explaining in part why DNA has emerged as the primary genetic material. Sugar, along with phosphate performs the structural role in nucleic acids. Generalized structural units of nucleic acids Generalized structural units of nucleic acids are indicated in the Scheme 1. Components of Nucleic acids: Deoxyribose (or ribose) Base Phosphoric acid Nucleoside Nucleotide Polynucleotide or Nucleic acid (DNA / RNA) Scheme 1: Generalized structural units of nucleic acid (A) Nucleosides The nucleosides are compounds in which nitrogenous bases (purines and pyrimidines) are conjugated to the pentose sugars (ribose or deoxyribose) by a β-N-glycosidic linkage. These consist of a base joined to a pentose sugar at position C1′. The sugar C1′ carbon atom is joined to the N1 atom of pyrimidine and the N9 atom of purine. This represents a β-N- glycosidic bond. Thus, the purine nucleosides are N-9 glycosides and the pyrimidine nucleosides are N-1 glycosides. These are stable in alkali. The purine nucleosides are readily hydrolyzed by acid whereas pyrimidine nucleosides are hydrolyzed only after prolonged treatment with concentrated acid. The nucleosides are generally named for the particular purine and pyrimidine present. Nucleosides possessing ribose are called ribonucleosides (riboside) and those containing deoxyribose are called deoxyribonucleosides (deoxyriboside). The nomenclature of nucleosides differs from that of the bases. 5 In case of pseudouridine, which is otherwise identical to uracil, differs in the point of attachment to the ribose to the base. In case of pseudouridine, base is attached to sugar through C5 of base as opposed to that in case of uridine, where the attachment of base to sugar is through N1 (structure described later in section of tRNA). Two nucleoside analogues, 3′-azidodeoxythymidine (AZT) and 2′, 3′-dideoxycytidine (DDC), have found therapeutic use for the treatment of acquired immune deficiency syndrome (AIDS) patients. (B) Nucleotides or Nucleoside 5’-triphosphates These are phosphate esters of nucleosides i.e. nucleosides form nucleotides by joining with phosphoric acid. Esterification can occur at any free hydroxyl group, but is most common at the 5′ and 3′ positions in sugars. The phosphate residues are joined to the sugar ring by a phosphomonoester bond and several phosphate groups can be joined in series by phosphoanhydride bonds. These occur either in the free form or as subunits in nucleic acids. The phosphate is always esterified to the sugar moiety. The trivial names of purine nucleosides end with the suffix –sine, and those of pyrimidine nucleosides end with suffix –dine. In addition to their role as structural components of nucleic acids, nucleotides also participate in a number of other functions as described below: Energy carriers: Nucleotides represent energy rich compounds that drive metabolic process, especially biosynthetic, in all cells. Hydrolysis of nucleoside triphosphate provides the chemical energy to drive a wide variety of cellular reactions. ATP is the most widely used for this purpose. UTP, GTP, CTP are also used. Nucleoside triphosphate also serves as the activated precursors of DNA and RNA synthesis. The hydrolysis of ester linkage (between ribose and α-phosphate) yields about 14 kJ / mol under standard conditions, whereas hydrolysis of each anhydride bond (between α-β and β-γ phosphates) yields about 30 kJ / mol. ATP hydrolysis often plays an important thermodynamic role in biosynthesis. Enzyme cofactors: Many enzyme cofactors include adenosine in their structure, e.g., NAD, NADP, FAD. Chemical messengers: Some nucleotides act as regulatory molecules and serve as chemical signals or secondary messengers, key links in cellular systems that respond to hormones and other extracellular stimuli and lead to adaptive changes in cells interior. Two hydroxyl groups can be esterified by the same phosphate moiety to generate a cyclic AMP (cAMP, adenosine 3’-5’ cyclic phosphate) or cyclic GMP (cGMP, guanosine 3’-5’ cyclic phosphate).
Details
-
File Typepdf
-
Upload Time-
-
Content LanguagesEnglish
-
Upload UserAnonymous/Not logged-in
-
File Pages39 Page
-
File Size-