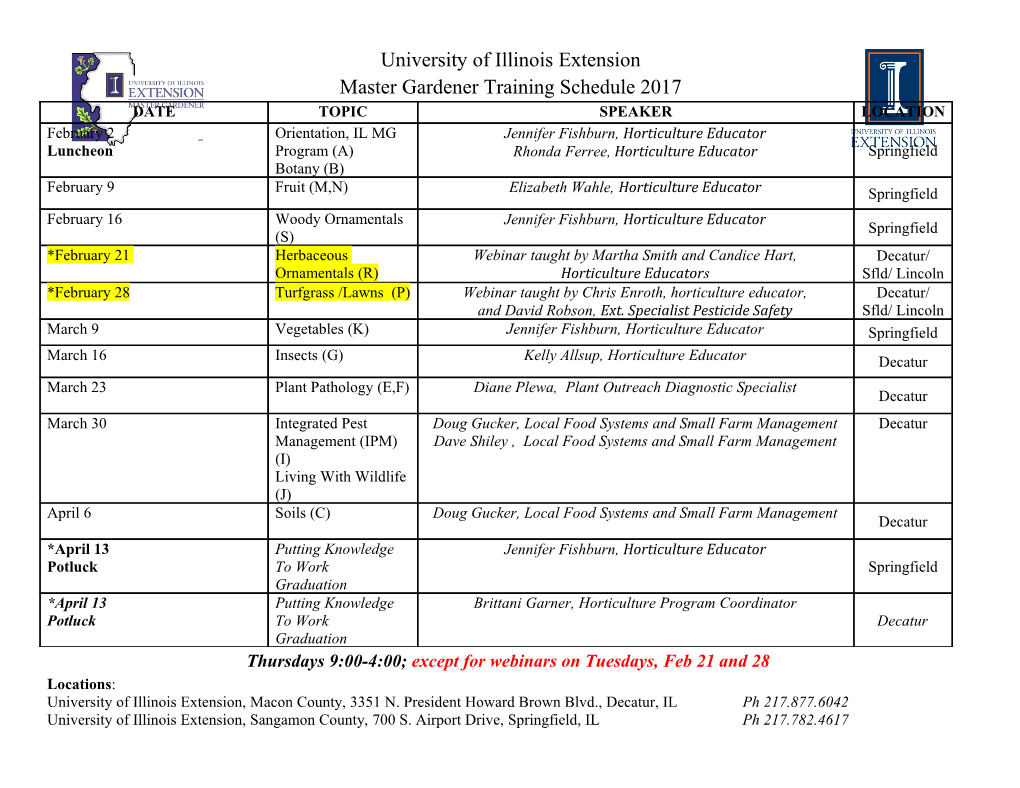
Appl Microbiol Biotechnol (2015) 99:6617–6642 DOI 10.1007/s00253-015-6699-6 MINI-REVIEW The substrate tolerance of alcohol oxidases Mathias Pickl2 & Michael Fuchs2 & Silvia M. Glueck1,2 & Kurt Faber2 Received: 7 April 2015 /Revised: 10 May 2015 /Accepted: 15 May 2015 /Published online: 8 July 2015 # The Author(s) 2015. This article is published with open access at Springerlink.com Abstract Alcohols are a rich source of compounds from re- Introduction newable sources, but they have to be activated in order to allow the modification of their carbon backbone. The latter Oxidation represents a fundamental reaction in nature can be achieved via oxidation to the corresponding aldehydes (Hollmann et al. 2011; Turner 2011), and oxidases are a prom- or ketones. As an alternative to (thermodynamically inent subclass of redox enzymes, which use oxygen either as disfavoured) nicotinamide-dependent alcohol dehydroge- oxidant or as electron acceptor. This property made them par- nases, alcohol oxidases make use of molecular oxygen but ticularly attractive for the production of chemicals their application is under-represented in synthetic biotransfor- (Vennestrom et al. 2010). In this context, the oxidation of mations. In this review, the mechanism of copper-containing alcohols is an important transformation in synthetic chemistry, and flavoprotein alcohol oxidases is discussed in view of their which allows to introduce carbonyl groups, which represent ability to accept electronically activated or non-activated alco- excellent acceptors for C-, N-, O-andS-nucleophiles and hols and their propensity towards over-oxidation of aldehydes thereby allows the extension of a given carbon backbone. yielding carboxylic acids. In order to facilitate the selection of Consequently, a large number of protocols has been devel- the optimal enzyme for a given biocatalytic application, the oped, which depend on (i) transition metals in stoichiometric substrate tolerance of alcohol oxidases is compiled and (e.g. Cr, Mn) or catalytic amounts (e.g. Ru, Fe), (ii) metal-free discussed: Substrates are classified into groups (non-activated oxidations according to Swern or Pfitzner-Moffat (Pfitzner prim-andsec-alcohols; activated allylic, cinnamic and ben- and Moffatt 1963; Omura and Swern 1978), (iii) molecular zylic alcohols; hydroxy acids; sugar alcohols; nucleotide al- oxygen as oxidant (Tojo and Fernández 2006) and more re- cohols; sterols) together with suitable alcohol oxidases, their cently, (iv) organocatalysts, such as TEMPO (Wertz and microbial source, relative activities and (stereo)selectivities. Studer 2013). In a related fashion, alcohol oxidases convert primary and secondary alcohols to aldehydes and ketones, respectively. Keywords Oxidation Biocatalysis Alcohol oxidase During this reaction, molecular oxygen is reduced to hydro- . Substrate tolerance Flavoprotein Cu-containing oxidase gen peroxide. In order to avoid enzyme deactivation, a cata- lase is usually employed, particularly on preparative scale. For Electronic supplementary material The online version of this article (doi:10.1007/s00253-015-6699-6) contains supplementary material, screening purposes, a spectrophotometric assay based on which is available to authorized users. horse radish peroxidase (HRP) together with a suitable artifi- cial electron acceptor, such as 2,2′-azino-bis(3- * Kurt Faber ethylbenzthiazoline-6-sulfonic acid) (ABTS) may be [email protected] employed (Scheme 1). The ABTS-radical generated shifts its absorption maximum (Baron et al. 1994; Uwajima and Terada 1 Austrian Centre of Industrial Biotechnology (ACIB GmbH), 1980). Petersgasse 14, 8010 Graz, Austria Although cofactor-lacking oxidases are reported (Fetzner 2 Department of Chemistry, Organic & Bioorganic Chemistry, and Steiner 2010), commonly used alcohol oxidases depend University of Graz, Heinrichstrasse 28, A-8010 Graz, Austria on flavin (Macheroux et al. 2011; Dijkman et al. 2013)ora 6618 Appl Microbiol Biotechnol (2015) 99:6617–6642 OH O alcohol oxidase stabilised oxocarbenium cation, which upon expulsion of H+ R1 R2 R1 R2 furnishes the carboxylic acid. In contrast, hydride abstraction Catalase or 1 from the carbonyl form would lead to a highly unstable O2 H2O2 H2O + /2 O2 HRP/ABTS (hypothetical) acylium cation, which would be quenched by Scheme 1 Biocatalytic oxidation of alcohols using an alcohol oxidase a water molecule (Scheme 3). This mechanism for over-oxidation has been proposed for choline oxidase (CHO), whose natural role is the formation of metal (Cu) as a cofactor (Whittaker 2003), which mediates the N-trimethylammonium glycine (‘betaine’) from choline via electron transfer. In flavoprotein oxidases, the oxidation pro- the aldehyde hydrate through a two-step oxidation (Scheme 4) ceeds via two half reactions, where the alcohol is first oxidised (Rungsrisuriyachai and Gadda 2008). by a two-electron transfer during the reductive half reaction, The over-oxidation of alcohols to carboxylic acids has been yielding reduced flavin. The oxidised flavin is regenerated by observed not only for choline oxidase but also for other fla- a stepwise single-electron transfer via the oxidative half voprotein oxidases, such as alditol oxidase (AldO), aryl alco- reaction, which requires triplet oxygen, as it is a spin- hol oxidase (AAO), hydroxymethyl furfuryl oxidase forbidden reaction. Hence, di-oxygen acts as single-electron (HMFO), hexose oxidase (HOX, Dbv29), isoamyl alcohol −· acceptor and forms superoxide (O2 ), stabilised by a positive- oxidase (IAO) or short- and long-chain alcohol oxidases ly charged histidine residue (Dijkman et al. 2013; Wongnate (SCAOs, LCAOs). Labelling studies proved the existence of et al. 2014). Another single-electron transfer yields a covalent the aldehyde hydrate as intermediate (Van Hellemond et al. hydroperoxy flavin intermediate, which eliminates hydrogen 2009), and for AAO, which naturally oxidises benzylic alco- peroxide and re-forms oxidised flavin (Scheme 2) (Gadda hols, NMR studies revealed that the gem-diol intermediate 2012). The highly unstable C4a-hydroperoxyflavin intermedi- was favoured (Ferreira et al. 2010)(Scheme3). ate has only been detected for pyranose oxidase (P2O) Structurally, most of the flavoprotein oxidases either be- (Mattevi 2006; Chaiyen et al. 2012; Wongnate and Chaiyen long to the glucose-methanol-choline (GMC) oxidase or the 2013). vanillyl alcohol oxidase (VAO) family. Both families have a The oxidation of primary alcohols catalysed by flavopro- flavin present in the active site where the binding domain and tein oxidases does not necessarily stop at the aldehyde stage, the binding mode of the flavin differ. In case of VAO, the but may further proceed to the corresponding carboxylic acid. flavin is covalently linked to a histidine, cysteine or tyrosine This second oxidation step is mechanistically less investigat- residue, while in the GMC family, the majority of the enzymes ed, but it is obvious that the actual substrate is the aldehyde contain a dissociable flavin adenine dinucleotide (FAD) moi- hydrate (gem-diol), rather than its carbonyl form, because hy- ety. In P2O or CHO, a covalent linkage was found. The active dride abstraction from the former yields a doubly resonance- sites and consequently the substrate scope of these enzymes Scheme 2 Catalytic cycle of flavin-containing alcohol oxidases Appl Microbiol Biotechnol (2015) 99:6617–6642 6619 O - [H-] OH O RC RCO RCO H2O H R1 R2 R1 R2 acylium cation (not formed) H+ carbonyl form reductive half reaction O RC H O + Cu2+ O Tyr Cu+ O Tyr 2 H OH H S S OH - OH - [H ] OH OH Cys + Cys RCOH RC RC RC H O OH OH H2O2 2 H OH hydrate form resonance-stabilised carbenium ion oxidative half reaction Scheme 3 Aldehyde oxidation via the hydrate intermediate Cu2+ O Tyr Cu2+ O Tyr O H HO S show high variance (Fraaije et al. 1998a; Kiess et al. 1998; S O OH Cys Leferink et al. 2008; Dijkman et al. 2013). Cys Another redox cofactor found in alcohol oxidases, such as Scheme 5 Catalytic cycle of copper-containing oxidases galactose oxidase (GOase), is the transition metal copper, whose role in catalysis is well described in several reviews size and/or polarity (Turner 2011). In fungi, extracellular al- (Ridge et al. 2008; Guengerich 2013). Since only a single cohol oxidases produce hydrogen peroxide (needed for lignin copper(I) ion is found in the active site, it seems surprising degradation by peroxidases) by oxidation of cinnamyl alco- that a two-electron transfer can occur. Detailed investigations hols (e.g. coniferyl, coumaryl and sinapyl alcohol). Further- revealed that the latter proceeds via two consecutive single- more, hydrogen peroxide acts as antibiotic in the rhizosphere electron transfer steps. Thus, abstraction of the first electron to protect roots (Monti et al. 2011). As an alternative to alco- by Cu2+ yields Cu+, which transfers its electron onto a tyrosine hol oxidases, NAD(P)+-dependent alcohol dehydrogenases residue, which forms a transient radical anion (Monti et al. provide a well-investigated enzyme platform for the oxidation 2011). The latter is stabilised by a rare covalent thioether of prim-andsec-alcohol functionalities. Although these en- bridge with an adjacent cysteine (Ito et al. 1991). The second zymes are more abundant than alcohol oxidases, the equilib- electron transfer yields a Cu+-tyrosine radical. From this, ox- rium for oxidation is strongly disfavored but can be overcome ygen accepts two electrons (Wang 1998; Whittaker 2003) by NAD(P)+ recycling (Hollmann et al. 2011).
Details
-
File Typepdf
-
Upload Time-
-
Content LanguagesEnglish
-
Upload UserAnonymous/Not logged-in
-
File Pages26 Page
-
File Size-