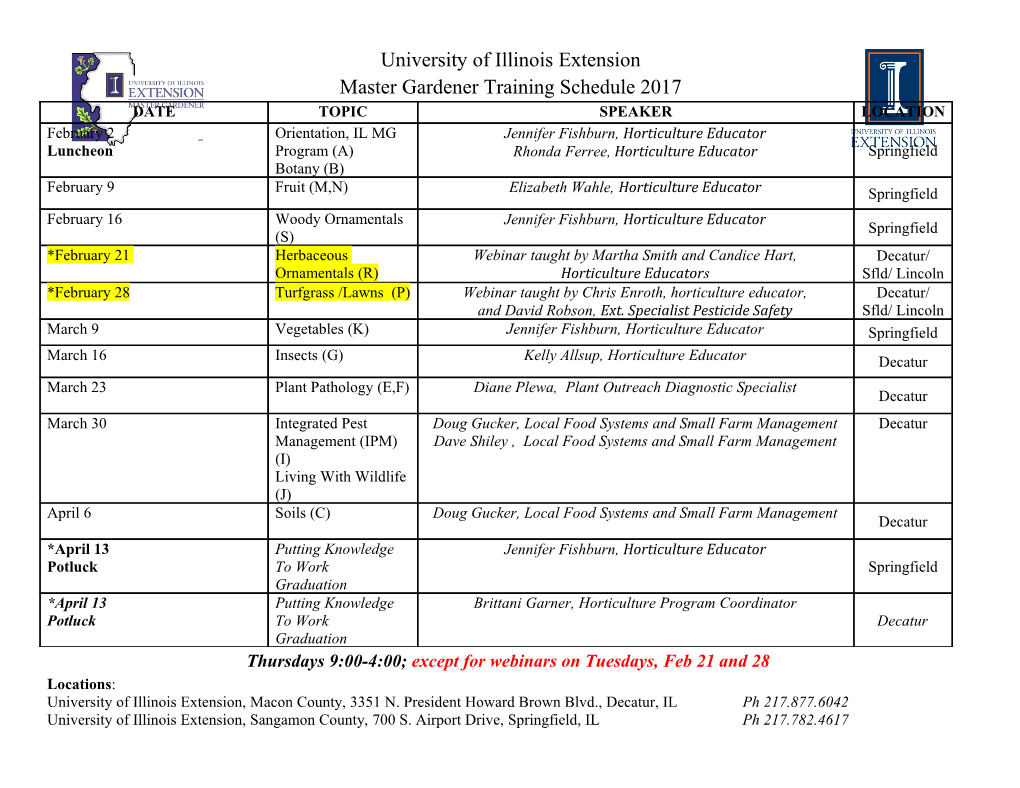
Seton Hall University eRepository @ Seton Hall Seton Hall University Dissertations and Theses Seton Hall University Dissertations and Theses (ETDs) 2010 Synthesis of Novel Glycosylidene-Based Quinolines Peter H. Dobbelaar Seton Hall University Follow this and additional works at: https://scholarship.shu.edu/dissertations Part of the Organic Chemistry Commons Recommended Citation Dobbelaar, Peter H., "Synthesis of Novel Glycosylidene-Based Quinolines" (2010). Seton Hall University Dissertations and Theses (ETDs). 1483. https://scholarship.shu.edu/dissertations/1483 Synthesis of Novel Glycosylidene-Based Quinolines A Dissertation In The Department of Chemistry and Biochemistry submitted to the faculty of the Graduate School of Arts and Sciences in partial fulfillment of the requirements for the degree of Doctor of Philosophy In Chemistry Seton Hall University by Peter H. Dobbelaar May 2010 We certify that we have read this thesis and that in our opinion it is adequate in scientific scope and quality as a dissertation for the degree of Doctor of Philosophy. APPROVED Signature 3.Q> Dr. Cecilia H. Marzabadi, Advisor -TI-6 Ity, Reader and Department Chair Approved for the Department of Chemistry and Biochemistry Acknowledgements I owe a debt of gratitude to my graduate advisor, Dr. Cecilia H. Marzabadi, for her guidance during my studies at Seton Hall University. In addition, I am also grateful for the support of my supervisors, Maryadele J. O'Neil and Patricia E. Heckelman, both of The Merck Index, as well as Dr. Cherie B. Koch, my previous supervisor and a former Merck Index editor. Their backing was pivotal in obtaining financial assistance through The Merck Research Laboratories Doctoral Program. I thank the past and present leadership of Merck's Department of Medicinal Chemistry, Rahway, NJ for the permission to use their laboratory facilities. I also gratefully acknowledge my Merck sponsor, Dr. Vincent J. Colandrea of Merck Research Laboratories, for his willingness to serve as a mentor and aid in my educational and scientific development. I deeply appreciate the expertise of the following scientists of Merck Research Laboratories: Drs. George A. Doss and Mikhail Reibarkh for both NMR and molecular modeling assistance, Dr. Charles W. Ross I11 and Kithsiri B. Herath for mass spectrometry support, Eric C. Streckfuss and Craig K. Esser for chromatography suggestions, Dr. R. Scott Hoerrner for assistance with high pressure hydrogenolysis experiments, Dorothy A. Levorse for salt stoichiometry and pKa analysis, and Dr. Yan Lin for discussions involving sugar chemistry. I acknowledge Rita A. Nacchio-Wells and the Merck library staff for the prompt retrieval of non-electronic references. I am grateful to the National Cancer Institute for testing several compounds in their in vilro cancer screens. I also thank my friends, Patrick J. Pollard, J.D. and Chris L. Franklin, for their particularly useful discussions as well as Jeremy M. West for technical support. Finally, as I have become iii increasingly driven to complete this project, I now understand why so many authors acknowledge the patient understanding of family. I thank my mother, Janet C. Dobbelaar, for encouraging me to continue my education. I dedicate this work to the memory of my grandparents, Janet and Robert 0. Schlenker. Inspiration Spoken by a True Leader - A Leader of Character, Competence, and Courage "Hang tough! Never, ever give up." Major Richard D. Winters, Ret. Easy Company, 506" Parachute Infantry Regiment 10 1" Airborne Division, US Army Band of Brothers, WWII Table of Contents Acknowledgements iii Inspiration v List of Tables ix List of Schemes xi .. List of Figures Xlll Abstract xv Chapter 1. The Properties and Biological Significance of Carbohydrates 1. Overview and Historical Perspective 2. Physical and Chemical Properties 3. Biological Relevance 4. Therapeutic Applications 5. Glycosidase Inhibitors 6. Industrial Importance of Carbohydrates 7. Summary Chapter 2. Synthesis of Glucose-Derived Quinolines 1. Introduction 2. Background a. Glycals and Spiroanellation Chemistry b. Synthesis of Quinolines Via the Povarov Reaction 3. Results a. Synthesis of Starting Materials b. Catalyst Screening c. Structural Determination d. Reaction Optimization e. Evaluation of Substituent Effects f. Deprotection Attempts 4. Summary Chapter 3. Reaction Scope Expansion 1. Introduction 2. Background a. Biological Relevance of Arabinose and Galactose b. Scope Expansion Rationale c. Reaction Stereochemistry 3. Results a. exo-Glycal Dienophiles b. Azadienes c. Further Transformations of Sugar-Derived Quinolines d. Stereochemistry of the Povarov Addition Reaction 4. Summary vii Chapter 4. Biological Evaluation of Selected Compounds 1. Introduction 2. Background a. Physiochemical Properties b. Anticancer Screening 3. Results a. Physiochemical Properties Assessment b. in vitro Anticancer Activity 4. Summary Chapter 5. Conclusions 1. Summary 2. Experimentals 3. References 4. Appendix (NMR spectra) viii List of Tables Table 2.0. Catalyst and reaction condition screening. Table 2.1. Spectral analysis for the spiroanellated tetrahydroquinoline 87Major. Table 2.2. Spectral analysis for the spiroanellated tetrahydroquinoline 87Minor. Table 2.3. Spectral analysis for the open-ring glycosylidene-derived quinoline 88a. Table 2.4. Screening of Povarov reaction conditions involving catalysis by Sc(0Tfh at various temperatures. Table 2.5. Reaction optimization experiments involving addition of molecular sieves and/or 2,6-di-[err-butyl-4-methylpyridine92. Table 2.6. Reaction optimization experiments involving a microwave reactor. Table 2.7. Examination of the electronic effect ofpara-substituted, benzaldehyde- derived imines on the one-pot synthesis of glycosylidene-based quinolines. Table 2.8. Examination of the electronic effect ofpara-substituted, anilinederived imines on the one-pot synthesis of glycosylidene-based quinolines. Table 2.9. Hydrogen balloon debenzylation experiments. Table 2.10. High pressure, high temperature debenzylation experiments. Table 2.1 1. Transfer hydrogenation debenzylation experiments. Table 2.12. Debenzylation experiments utilizing chemical methods. Table 3.0. Synthesis of arabinose-derived quinolines. Table 3.1. Synthesis of galactose-derived quinolines. Table 3.2. Catalyst and reaction condition screening with azobenzene 100. Table 3.3. Quinoline N-methylation attempts. Table 3.4. Glycosylation attempts. Table 3.5. Molecular modeling calculations for both the observed and potential Povarov reaction products. Table 3.6. Stereoselective reaction condition screening. Table 4.0. Calculated physiochemical properties of novel glycosylidene-derived quinolines. Table 4.1. Results from the NCI60 human tumor cell line anticancer drug screen assay. List of Schemes Scheme 1.0. Chemical equations for the processes of (a) photosynthesis and (b) respiration. Scheme 2.0. Knapp's approach toward carbohydrate-fused thiazolines. Scheme 2.1. DeCastro and Marzabadi's synthesis of oxazoline-fused carbohydrates. Scheme 2.2. Talisman and Marzabadi's synthesis of carboethoxy cyclopropanated carbohydrates. Scheme 2.3. Somsak's synthesis of an analogue of 2-thiohydantocidin. Scheme 2.4. Synthesis of furanoid spironucleosides of oxazolidine-2-thiones and oxazinane-2-thiones. Scheme 2.5. Generalized Povarov reaction sequence. Scheme 2.6. Currently postulated, non-concerted mechanism for the Povarov reaction illustrated with BFj'Et20 as the Lewis acid catalyst. Scheme 2.7. Multicomponent reactions involving glycal addition reported by Jiminez and colleagues. Scheme 2.8. First reported Povarov reaction utilizing a glycal derivative as the activated alkene. Scheme 2.9. Preparation of quinoline 80. Scheme 2.10. Preparation of glucose-fused quinoline 82. Scheme 2.1 1. Synthesis of the essential exo-glycal intermediate. Scheme 2.12. Synthesis of benzanilines. Scheme 2.13. Benzyl ether deprotection of glucose-based quinoline 88a. Scheme 3.0. Ishanti and Kobayashi's stereoselective Povarov reaction. Scheme 3.1. Synthesis of the arabinose-derived exo-glycal. Scheme 3.2. Synthesis of the arabinose-spiroanellated tetrahydroquinoline. Scheme 3.3. Debenzylation of arabinose-based quinolines llOa and 110b. Scheme 3.4. Synthesis of the galactose-derived exo-glycal. Scheme 3.5. Debenzylation of galactose-based quinoline 115a. Scheme 3.6. Povarov reaction attempts with ethenyl exo-glycal 118. Scheme 3.7. Povarov reaction attempts with imine 99. Scheme 3.8. Formation of the hydrochloride salt of 93a. Scheme 3.9. Deprotection of spiroanellated tetrahydroquinoline 87Major. List of Figures Figure 1.O. Structural representations of D-glucose. Figure 1.1. Mutarotation of glucose in water at 3 1 "C. Figure 1.2. Equilibrium chair conversions of glucose. Figure 1.3. Practical illustration of the anomeric effect. Figure 1.4. Glucose-based storage carbohydrates. Figure 1.5. Repeating units of polysaccharide polymers used by organisms for structural support. Figure 1.6. Structural representations of (a) a generic nucleotide and (b) nucleotide sugars. Figure 1.7. Several carbohydrate-based therapeutics. Figure 1.8. Macrolide antibiotics. Figure 1.9. Anticancer and antiviral carbohydrate-based drugs. Figure 1.10. Generic illustration of the activity of a glycosidase enzyme. Figure 1.1 1. Carbohydrate-based glycosidase inhibitors. Figure 1.12. Azasugar glycosidase inhibitors. Figure 2.0. Carbohydrate-based diabetes drugs. Figure 2.1. Examples of carbohydrate-spiro heterocycles with glycosidase inhibitory activity. Figure
Details
-
File Typepdf
-
Upload Time-
-
Content LanguagesEnglish
-
Upload UserAnonymous/Not logged-in
-
File Pages224 Page
-
File Size-