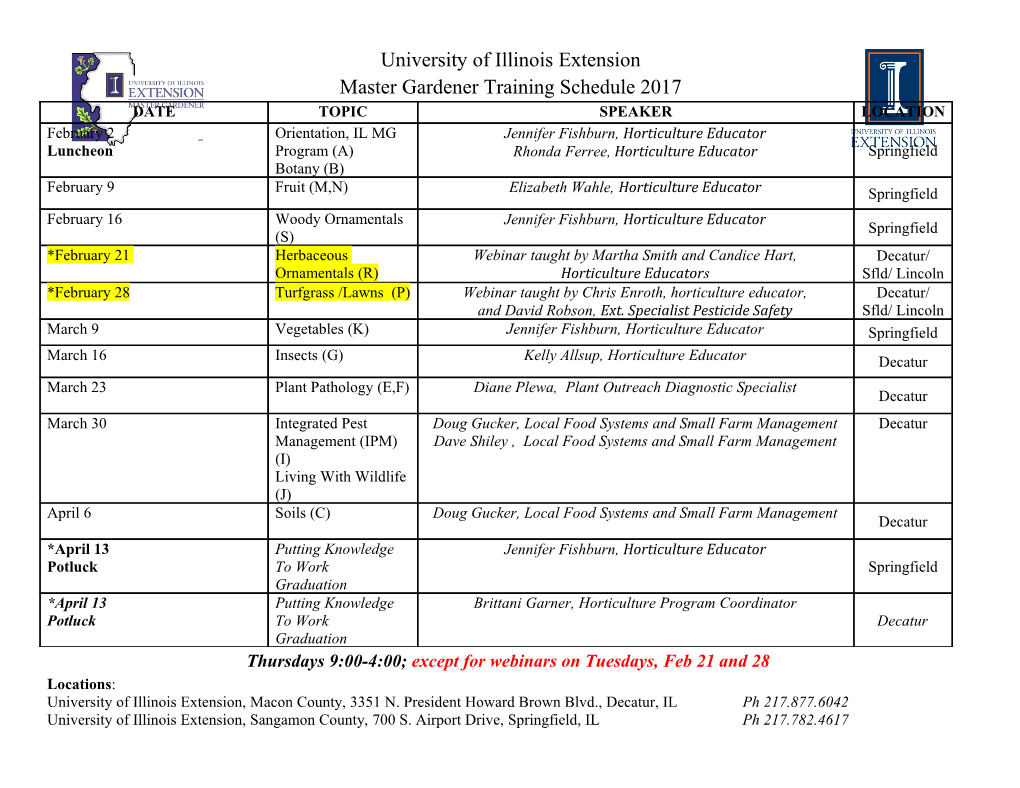
Chemical Geology 300-301 (2012) 123–132 Contents lists available at SciVerse ScienceDirect Chemical Geology journal homepage: www.elsevier.com/locate/chemgeo Isotopic and microbiological signatures of pyrite-driven denitrification in a sandy aquifer Yan-Chun Zhang a, Caroline P. Slomp a,⁎, Hans Peter Broers b,c,d, Benjamin Bostick e, Hilde F. Passier b, Michael E. Böttcher f,1, Enoma O. Omoregie a,g, Jonathan R. Lloyd g, David A. Polya g, Philippe Van Cappellen a,h,i a Department of Earth Sciences, Faculty of Geosciences, Utrecht University, Budapestlaan 4, 3584 CD Utrecht, The Netherlands b Deltares Research Institute, Subsurface and Groundwater, Utrecht, The Netherlands c TNO, Geological Survey of the Netherlands, Utrecht, The Netherlands d VU University Amsterdam, De Boelelaan 1085, 1081 HV Amsterdam, The Netherlands e Lamont-Doherty Earth Observatory of Columbia University, Palisades NY, USA f Max Planck Institute for Marine Microbiology, Bremen, Germany g School of Earth, Atmospheric and Environmental Sciences, University of Manchester, Manchester, UK h School of Earth and Atmospheric Sciences, Georgia Institute of Technology, Atlanta, USA i Department of Earth and Environmental Sciences, University of Waterloo, Ontario, Canada article info abstract Article history: Denitrification driven by pyrite oxidation can play a major role in the removal of nitrate from groundwater Received 11 September 2011 systems. As yet, limited information is available on the interactions between the micro-organisms and aque- Received in revised form 23 December 2011 ous and mineral phases in aquifers where pyrite oxidation is occurring. In this study, we examine the ground- Accepted 14 January 2012 water and sediment composition along a well-characterized redox gradient in a heavily nitrate-polluted Available online 31 January 2012 pyritic sandy aquifer in Oostrum (the Netherlands) to identify the sequence of steps involved in denitrifica- δ15 − δ18 − δ34 2 − δ18 2 − Editor: B. Sherwood Lollar tion coupled to pyrite oxidation. Multi-isotope analyses ( N-NO3 , O-NO3 , S-SO4 , O-SO4 and 34 δ Spyrite) confirm that pyrite is the main electron donor for denitrification at this location. Enrichment Keywords: factors derived from the observed changes in nitrate isotopic composition range from −2.0 to −10.9‰ for Denitrification ε15N and from −2.0 to −9.1‰ for ε18O. The isotopic data indicate that pyrite oxidation accounts for approx- Pyrite imately 70% of the sulfate present in the zone of denitrification. Solid-phase analyses confirm the presence of Groundwater pyrite- and organic matter-rich clay lenses in the subsurface at Oostrum. In addition, sulfur XANES and iron Isotopes 2 − XAS results suggest the presence of a series of intermediate sulfur species (elemental sulfur and SO3 ) that Microbiology may be produced during denitrification. Consistent with geochemical analysis, 16S rRNA gene sequencing Sediment revealed the presence of bacteria capable of sulfide oxidation coupled to nitrate reduction and that are tolerant to high aqueous metal concentrations. © 2012 Elsevier B.V. All rights reserved. 1. Introduction Pyrite oxidation leads to sulfate production and trace metal re- lease to the groundwater (Broers, 1998). This process can have a Nitrate is a common groundwater pollutant especially in regions major impact on local and regional water quality. Additional (inter- of intensive agriculture (Strebel et al., 1989). The major sources of ni- mediate) products may include nitrite and nitrous oxide (Appelo trate are manure, fertilizers, atmospheric deposition, soils and plants and Postma, 2005). Several studies have provided compelling evi- (Kendall et al., 2007). Denitrification is a natural microbial attenua- dence for the occurrence of nitrate reduction coupled to pyrite oxida- tion process (Korom, 1992) in which microorganisms use organic tion at field sites (Broers and Buijs, 1997; Pauwels et al., 1998; Postma matter or inorganic compounds as electron donors. When pyrite et al., 1991; Schwientek et al., 2008; Jørgensen et al., 2009; Tesoriero (FeS2) acts as the electron donor, the process can be represented by et al., 2000; Zhang et al., 2009). These studies further suggest that de- the following idealized overall reaction: nitrification with pyrite can be the dominant pathway of nitrate re- moval from groundwater, even when organic matter is present. ð Þþ − þ þ→ 2þ þ ð Þþ 2− þ ð Þ 5FeS2 pyrite 14NO3 4H 5Fe 7N2 g 10SO4 2H2O 1 Recent laboratory experiments with sediment from an agricultural area have confirmed the microbial nature of the process (Jørgensen et al., 2009). However, sulfate production in pyritic aquifers is often ⁎ Corresponding author. Tel.: +31 302535514; fax: +31 302535302. E-mail address: [email protected] (C.P. Slomp). found to be lower than predicted by Eq. (1) (e.g. (Postma et al., 1 Present address: Leibniz Institute for Baltic Sea Research, Warnemünde, Germany. 1991; Miotliński1, 2008; Zhang et al., 2009). This may indicate that 0009-2541/$ – see front matter © 2012 Elsevier B.V. All rights reserved. doi:10.1016/j.chemgeo.2012.01.024 124 Y.-C. Zhang et al. / Chemical Geology 300-301 (2012) 123–132 other processes are active, such as incomplete pyrite oxidation to el- emental sulfur (Zhang et al., 2009). An alternative explanation for the mismatch between the observed changes in groundwater nitrate and sulfate with depth is that inputs of nitrogen from agriculture have de- clined with time. As a consequence, the present-day input of nitrate 14.0 to the zone of pyrite oxidation may not directly correspond to the 15.01 amount of sulfate produced at depth. 5 .0 Stable isotope analysis is a powerful tool to identify the sources and sinks of bioactive compounds in natural environments, because the 16.0 sources may have characteristic isotopic signatures and the isotopes are often strongly fractionated during biogeochemical transformations. 38 15 − 34 2− This holds for the commonly studied δ N-NO3 and δ S-SO4 ,aswell 17.017. as for the oxygen isotope signatures of nitrate and sulfate. The simulta- 0 neous determination of stable isotope ratios of multiple elements may 40 significantly strengthen the interpretation in terms of provenance and 41 18.01 transformation processes (Böttcher et al., 1990, 2001; Deutsch et al., 8.0 2006; Schwientek et al., 2008; Pauwels et al., 2010). For example, 1km 15 − Böttcher and coworkers showed that groundwater δ N-NO3 and 18 − 42 19.0 Forest δ O-NO3 values in a catchment area with high nitrate input were con- sistent with denitrification coupled to pyrite oxidation (Böttcher et al., 20.0 Farmland 1990). Similarly, isotope data of groundwater sulfate and sediment Town 7649 sulfur compounds for a carbonate-containing organic-poor aquifer suggest that pyrite oxidation is the dominant source of sulfate in the Fig. 1. Well locations at Oostrum. Wells 40, 41 and 42 are located under farmland, groundwater system (Schwientek et al., 2008). whereas well 38 is located in a forested area. The arrow indicates the general ground- water flow direction (Zhang et al., 2009). Two sediment cores were drilled at a distance The latter study also illustrates the need for combined studies of of ~3 m west (Core 1) and ~200 m northeast (Core 2) of well 41. the groundwater and solid phase composition for a correct under- standing of spatial and temporal trends in microbially-mediated pro- cesses in aquifers. Within this context, detailed sediment speciation farmland, well 38 is located in a nearby forested area (Broers and analyses with techniques such as X-ray spectroscopy are of particular Buijs, 1997). The investigated aquifer is unconfined and has an ap- value because they allow potential solid phase intermediates of pyrite proximate thickness of 45 m. Impermeable clay layers at its bottom oxidation to be identified (Ziegler et al., 2009). Detailed microbial clearly define the base of the aquifer. The main aquifer is usually sit- analysis of aquifer sediments can provide complimentary information uated between 15 and 45 m depth and consists of coarse fluvial on biogeochemical processes by providing insight into the organisms sands of Pliocene age with, in the upper part, unconnected clay that mediate these processes. Such studies have been performed for lenses, which often have high organic matter contents. Across the heterotrophic and autotrophic denitrifiers in marine sediments and area, there are several faults, which are revealed by horizontal discon- laboratory incubations. For example, Brettar et al. (2006) identified tinuities in hydraulic head. The depth, thickness and grain size distri- autotrophic denitrifiers at the oxic-anoxic interface in the Baltic Sea bution of the intermediate clay layer is spatially variable, indeed, the (Brettar et al., 2006). Various laboratory studies suggest Thiobacillus clay is sometimes absent in parts of the area. Thin centimeter thick denitrificans is capable of coupling denitrification to pyrite oxidation lenses of clay are present in the top of the Pliocene aquifer, just (Jørgensen et al., 2009; Torrentó et al., 2010). However, studies iden- below the clay layer which separates the Pliocene and Pleistocene se- tifying the bacteria responsible for denitrification coupled to pyrite quence. The Pliocene sequence is overlain by ~10 m of coarse fluvial oxidation in aquifer sediments from field sites are not yet available. sands and gravels of Pleistocene age which were deposited by the 34 2− 18 2− Here, we combine multi-isotope analyses (i.e., δ S-SO4 , δ O-SO4 , Meuse river and ~5 m of eolian sand deposits. The groundwater 15 − 18 − 34 δ N-NO3 , δ O-NO3 , and sediment δ Spyrite) with microbial analysis table is about 3–5 m below the land surface. Groundwater sampling of the sediment (16S rRNA gene amplification and sequencing) and was carried out in 1996 and 2006 and is described in detail elsewhere detailed field data of the aqueous and solid phase geochemistry for a (Zhang et al., 2009). Here, we focus on the results for 2006.
Details
-
File Typepdf
-
Upload Time-
-
Content LanguagesEnglish
-
Upload UserAnonymous/Not logged-in
-
File Pages10 Page
-
File Size-