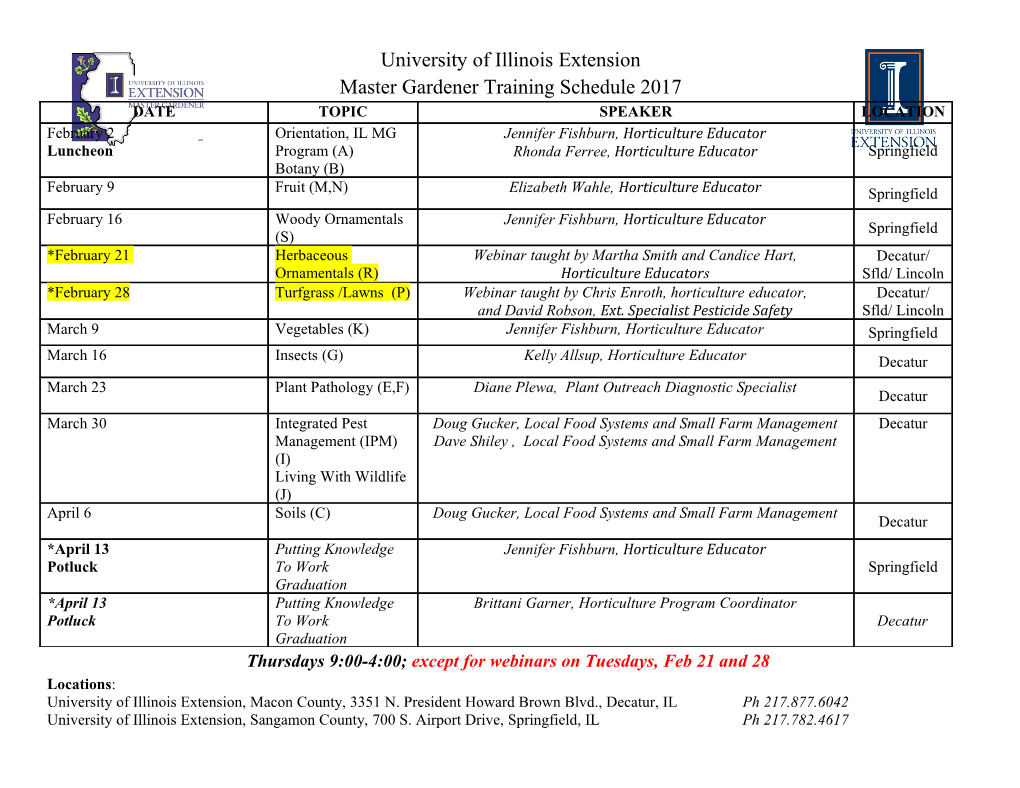
Lawrence Berkeley National Laboratory Recent Work Title Structural and spectroscopic characterization of an einsteinium complex. Permalink https://escholarship.org/uc/item/8gx712r6 Journal Nature, 590(7844) ISSN 0028-0836 Authors Carter, Korey P Shield, Katherine M Smith, Kurt F et al. Publication Date 2021-02-03 DOI 10.1038/s41586-020-03179-3 Peer reviewed eScholarship.org Powered by the California Digital Library University of California Article Structural and spectroscopic characterization of an einsteinium complex https://doi.org/10.1038/s41586-020-03179-3 Korey P. Carter1, Katherine M. Shield1,2, Kurt F. Smith1, Zachary R. Jones3, Jennifer N. Wacker3,4, Leticia Arnedo-Sanchez1, Tracy M. Mattox5, Liane M. Moreau1, Received: 3 July 2020 Karah E. Knope4, Stosh A. Kozimor3 ✉, Corwin H. Booth1 ✉ & Rebecca J. Abergel1,2 ✉ Accepted: 29 October 2020 Published online: xx xx xxxx The transplutonium elements (atomic numbers 95–103) are a group of metals that lie Check for updates at the edge of the periodic table. As a result, the patterns and trends used to predict and control the physics and chemistry for transition metals, main-group elements and lanthanides are less applicable to transplutonium elements. Furthermore, understanding the properties of these heavy elements has been restricted by their scarcity and high radioactivity. This is especially true for einsteinium (Es), the heaviest element on the periodic table that can currently be generated in quantities sufficient to enable classical macroscale studies1. Here we characterize a coordination complex of einsteinium, using less than 200 nanograms of 254Es (half-life of 275.7 days), with an organic hydroxypyridinone-based chelating ligand. Structural studies are used to determine the energy of the L3 absorption edge and a bond distance of einsteinium. Photophysical measurements reveal antenna sensitization of EsIII luminescence; they also reveal a hypsochromic shift on metal complexation, which had not previously been observed in lower-atomic-number actinide elements. These findings are indicative of an intermediate spin–orbit coupling scheme in which j–j coupling (whereby single-electron orbital angular momentum and spin are first coupled to form a total angular momentum, j) prevails over Russell–Saunders coupling. Together with previous actinide complexation studies2, our results highlight the need to continue studying the unusual behaviour of the actinide elements, especially those that are scarce and short-lived. Q1 The high radioactivity and scarcity of Es isotopes have precluded this optical measurements have also been reported1,11–14. Furthermore, ther- element from receiving the same attention as its preceding neigh- modynamic stability constants have been measured for a limited series − − 2− − Q2 bours within the actinide series, the bonding, electronic structure of common complexing agents (Cl , OH , SO4 and SCN ) and chelators and chemical properties of which are assumed to be between those (ethylenediaminetetraacetic acid and diethylenetriaminepentaacetic of the transition metals and the lanthanides1. This unusual chemical acid), using radioactive tracer methods1,15–18. Beyond this, the chemis- behaviour is often attributed to the emergence of the 5f orbital mani- try of Es is unexplored and so there is insufficient experimental data fold, large spin–orbit coupling and substantial relativistic effects3, and to validate theoretical predictions regarding structure and bonding. all these properties are known to increase across the actinide series4. One reason it is difficult to make accurate predictions for Es is because Contemporary worldwide availability of Es is restricted to small-scale of its location in the actinide series. For example, it is unclear which quantities (nano- to micrograms) of one of its two long-lived isotopes, oxidation states will be stable in numerous chemical environments 254 Es (half-life t1/2 = 275.7 days), the decay rate of which is roughly 300 because Es lies between larger minor actinides (Am, Cm, Bk and Cf) times faster than that of its longer-lived transplutonium neighbour, that favour the +3 oxidation state and smaller late actinides (Fm, Md, 249Cf (ref. 5). However, there are some examples that demonstrate how No and Lr), for which the +2 oxidation state becomes increasingly acces- the technical challenges associated with working with transplutonium sible3. Predicting changes in reactivity and electronic structureduring elements can be overcome, even for less accessible elements such as a chemical process is rarely possible for Es because—in comparison to Q3 Es. Highlights from the few previous Es studies include establishing other actinides—its Lewis acidity is large, its ionic radius is small and Q4 6 III 10 2 nuclear properties from Es isotopes , purifying Es from neighbour- it has a substantial number of unpaired 5f electrons (Es is (Rn) 5f ) . Q5 ing actinides7–9, and initial reporting of various ionization potentials Here we report how we overcame the challenges of working with Q6 Q7 and thermodynamic properties of Es1,10. A few simple inorganic com- small quantities of 254Es (less than 200 ng) to enable the characteriza- pounds—oxides and halides—of Es have been prepared. These com- tion of an Es coordination complex in solution and as a solid. We chose pounds were characterized in terms of their unit-cell parameters; some the octadentate hydroxypyridinone ligand 3,4,3-LI(1,2-HOPO) (HOPO; 1Chemical Sciences Division, Lawrence Berkeley National Laboratory, Berkeley, CA, USA. 2Department of Nuclear Engineering, University of California, Berkeley, CA, USA. 3Los Alamos National Laboratory, Los Alamos, NM, USA. 4Department of Chemistry, Georgetown University, Washington, DC, USA. 5Molecular Foundry, Lawrence Berkeley National Laboratory, Berkeley, CA, USA. ✉e-mail: [email protected]; [email protected]; [email protected] Nature | www.nature.com | 1 Article OH O O OH N O N N O N N N H N N O O O O O O O NH Es O O O 4H+ N H N O O N N 3+ N O N N O Es H OH O O OH 3,4,3-LI(1,2-HOPO) [EsIII(3,4,3-LI(1,2-HOPO))]– Fig. 1 | The organic ligand 3,4,3-LI(1,2-HOPO) forms an EsIII coordination attached to a spermine scaffold (blue) through amide linkages. Eight complex, enabling structural and spectroscopic studies. The octadentate metal-binding O atoms (red) serve as the first coordination sphere around EsIII chelator is composed of four 1-hydroxy-pyridin-2-one metal binding units on ligand deprotonation and metal complexation. Fig. 1) as the complexing agent, because of its well established chelation For XAS studies, 254Es (17 μM, 40 μl) was complexed with HOPO at properties with transition metals and f elements, and its propensity to a 1:10 metal-to-ligand ratio in aqueous solution buffered at pH 7–8 act as a luminescence sensitization antenna. The latter characteristic to ensure full ligand deprotonation and the formation of a single Es enabled the detection of the luminescence spectrum of Es, even with less species, [EsIII(HOPO)]− (Fig. 1). The solution was then dropcast into a than 100 ng available for study. Luminescence measurements strongly 3D-printed sample holder. The resulting solid residue was triply con- suggest that Es was in the +3 oxidation state when chelated by HOPO. tained and the sample was cooled with liquid nitrogen (about 77 K), These optical results complement structural characterization of the before the L3-edge XAS spectrum of Es was collected in fluorescence corresponding complex using trace-level X-ray absorption spectroscopy mode. Balancing moderate brightness from the Stanford Synchrotron (XAS) measurements at the Stanford Synchrotron Radiation Lightsource. Radiation Lightsource with the trace-level detection capabilities of the ab 2.0 5.0 1.5 2.5 ) –3 1.0 ) (Å 0 k ( χ 3 k Normalized absorption 0.5 –2.5 Unltered Filtered Fit 0 –5.0 20,360 20,380 20,400 20,420 20,440 2 34567 E (eV) k (Å–1) cd 5.0 2.50 2.5 2.45 Cf Cm ) Am –4 Å 0 2.40 )| ( r ( χ | Es –2.5 2.35 Amplitude M–O interatomic distance (Å) Real part Fit –5.0 2.30 01234 0.86 0.87 0.880.890.90 r (Å) (Ionic radius)–1 (Å–1) Fig. 2 | The structural features of the [EsIII(HOPO)]− coordination complex deviation of the mean between individual traces. The full data range for the raw were probed using element-specific L3-edge XAS at 77 K. a–c, XANES data is shown in Extended Data Fig. 2. d, The Es–O bond distance was validated spectrum (a), EXAFS data and fit (b), and Fourier transform of the k-space data against bond lengths determined from previously characterized [MIII(HOPO)]− and fit (c) for solid residue of [EsIII(HOPO)]−. E is the incident photon energy, (M = Am, Cm, Cf) complexes, with a coordination number of 9, highlighting a k3χ(k) is the EXAFS function and r represents the scattering distance (not substantially shorter M–O bond in the Es complex2. Ionic radii taken from ref. 26. phase-corrected). Fits were obtained as described in Methods. Data were Error bars are based on a profiling method28 from the fit, together with an transformed between 2.5 Å−1 and 6.6 Å−1 using a Gaussian window with a width of estimate of probable systematic error from the limited k range. 0.3 Å−1. Error bars for the raw, unfiltered data are estimated as the standard 2 | Nature | www.nature.com ab1 35,000 S1 30,000 0.75 25,000 T1 ) –1 m 20,000 0.50 15,000 Energy (c 5 I7 10,000 5I 0.25 5 Normalized emission intensity (a.u.) 5,000 0 5 0 S0 I8 III 11,000 10,000 9,000 8,000 7,000 3,4,3-LI Es (aq) (1,2-HOPO) Wavenumber (cm–1) Fig.
Details
-
File Typepdf
-
Upload Time-
-
Content LanguagesEnglish
-
Upload UserAnonymous/Not logged-in
-
File Pages20 Page
-
File Size-